J Biomed 2017; 2:12-19. doi:10.7150/jbm.17059 This volume Cite
Research Paper
Supercontinuum source in the investigation of laser-tissue interactions: “ex vivo” study
1. Group of Applied ElectroMagnetic, Information Engineering Department, University of Parma, Viale Parco Area delle Scienze 181/A 43124 Parma, Italy.
2. Micoralis Laboratory, Faculty of Dentistry, University of Nice, Pôle Universitaire St Jean d'Angély 24, Avenue des Diables Bleus 06357, Nice Cedex 4, France.
Abstract
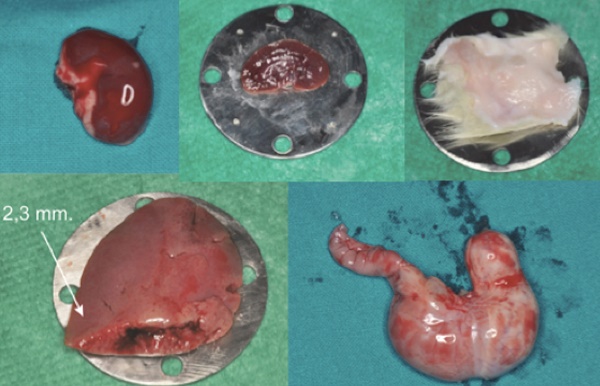
One of the main difficulties met by clinicians in their daily practice is the choice of the proper laser wavelength to obtain the best results from their treatments with minimal discomfort of the patients. This “ex vivo” study, based on the employment of a supercontinuum source, was performed with the purpose of defining the amount of energy transmitted through different animal tissues by wavelengths ranging from 350 up to 1700 nm. Supercontinuum is a particular light which combines some properties of the lamp light with the collimation beam of the laser. The transmission amount may help clinicians understand which wavelengths have no interaction with a particular tissue, thus determining which lasers are less useful and effective in the different clinical situations and locations. The experiments demonstrated the absence of transmitted energy in two portions of the spectrum, one (350-600 nm) in the visible part of the “therapeutic window” and one in the infra-red portion of the spectrum (1300-1700). Moving to clinical practice, this study suggests that using the visible (blue and green) and the far infra-red (around 1500 nm) lasers in surgery and LLLT is ideal.
Keywords: supercontinuum laser, transmission, laser-tissue interaction, ex vivo.
Background
The first applications of lasers in medicine was in the field of dermatology by Leon Goldman who described the removal of lesions such as melanomas and “port wine” stains from the skin [1].
Later on, medical laser applications increased more and more, including surgery, gynaecology, dentistry and neurology [2-4]. Then with the introduction of PDT (Photodynamic Therapy) and LLLT (Low Level Laser Therapy) in medicine, new therapeutic possibilities were offered to clinicians [5, 6]. However, with the appearance of new wavelengths on the market, physicians needed to determine the most effective laser for their treatments.
Authors compared the performances of different laser wavelengths in two different studies on animal models [7, 8] and they concluded that results are in function of the irradiated tissue features, particularly of the presence of chromophores (water, keratin, haemoglobin, etc.).
The literature analysis demonstrates that even in the field of LLLT, there is no concert about the choice of laser wavelength. Some studies indicate those in the infrared portion of the spectrum [9] as the most proper, but others suggest those in the visible, such as red [10] and green [11], while great interest was recently found in blue light utilisation [12].
From the perspective of laser-tissue interactions, the effect of a beam interaction with the target may be the reflection and refraction, the scattering, the absorption and the transmission [13] and the final outcome is the result of the optic characteristics of the processed tissue (reflection, scattering and absorption coefficients) [14] and of the laser parameters used (wavelength, operation mode, pulse duration, power density and Fluence) [15]. In clinics, while refraction has the main role in the interaction with transparent media, such as the cornea, it is not easy to quantify with the opaque media because of the great amount of absorption and scattering [16]. The laser beam passes through the target tissue, generating multiple scattering processes and finally becoming a broad diffuse beam [17]. While reflection is not influenced by the wavelength because it depends only on the angulation of the beam and the target characteristics, scattering is strictly related to wavelength (inversely proportional to its fourth power): therefore, a UV beam results more scattered than an IR [16].
When electro-magnetic radiation is absorbed in a medium, its intensity is weakened by the passage through the medium itself because of an incomplete transformation of energy into heat (molecular movement); the medium capacity to absorb radiation is in function with several factors, such as the electronic structure of beam wavelength, the thickness of the medium and the temperature [17].
With a laser, which is monochromatic, exactly the contrary happens: when a beam of I0 intensity interacts with a liquid, some of its energy is absorbed by the solution, and this is the reason why the intensity of the transmitted beam is < I0. Even if the particles scattering in the solution and the reflection at the interface cause a loss of intensity, the beam is mainly absorbed by the solution and the relationship between I and I0 depends on the path length of the absorbing solution. c. Lambert and Beer defined the relationship between all these factors in the law which is called by their names [18].
The most important optic parameter used to choose the best wavelength in clinical procedures is represented by the depth of its penetration, defined as the depth at which the intensity of the radiation reduces to 37% of its highest at the surface of the irradiated target. This parameter is mostly dependent on the wavelength [19]: in the red and near infrared portions, it may be very high, due to the absorption by water, whereas UV and visible beams are best absorbed by proteins and coloured particles [20].
The only evaluation of the transmission amount does not indicate the respective contribution of scattered and absorbed energy; however, it may establish what wavelengths do not interact with the target media. [21].
The purpose of this “ex vivo” study was to establish, with a supercontinuum source, the quantity of the transmitted energy through each tissue by each wavelength.
The supercontinuum light, discovered by Alfano in the 1970s, is the topic of a great number of studies and research [22]; it is generated by provoking a broadband polarization in a dielectric medium by means of a high energy source by a high power laser [23]: in this way, it combines the broadband characteristics of a lamp with coherence and high brightness of laser [24]. (Fig. 1).
Typical supercontinuum spectrum, the blue line shows the spectrum of the pump source and the red line the resulting supercontinuum spectrum generation.
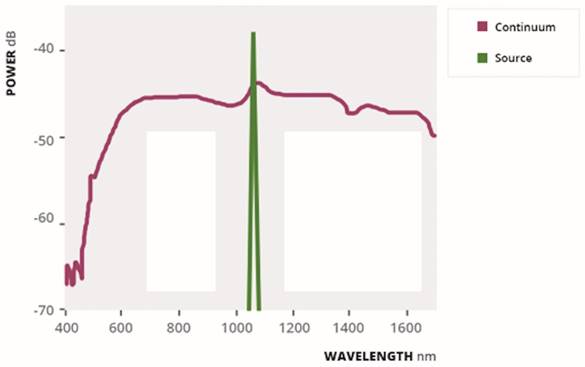
Different kinds of tissue collected by the Sprague Dawley rats.
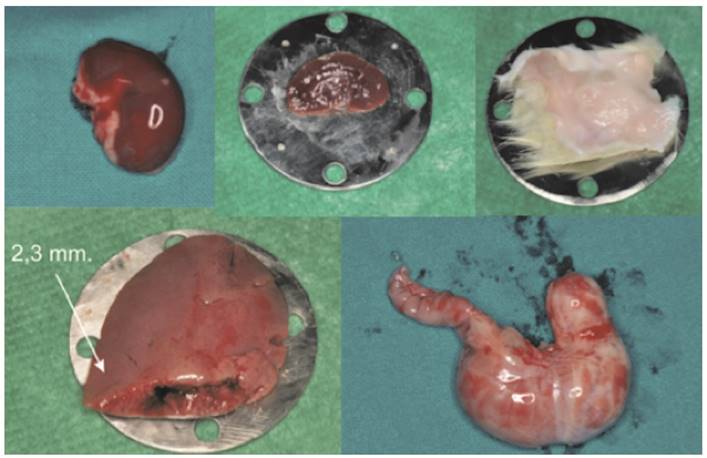
Methods
All the samples used for the tests were obtained by control subjects among Sprague Dawley rats of a study performed in the Department of Dentistry, and the study was planned according to the Declaration of Helsinki. It was approved by the Veterinary Sciences Committee for Ethics of Parma University and the Italian Ministry of Public Health.
At the time of sacrifice, different organs were taken away and soon utilised for the study.
The different tissues used and their thicknesses are shown in Fig. 2 and Table 1.
The instrument utilised for the tests was the SuperK Compact (NKT Photonics, Denmark), which generates a white light from a 1064 nm pulsed laser emitting 2 ns pulses. The beam is focused on a 1 μm diameter optic fiber core, thus generating non-linear effects due to the reduced size of the fiber and finally producing an emission of broadband radiation ranging from 500 to 2400 nm.
After passing through the sample, the beam was focused on the core of a 50 μm diameter multimode optic fiber by a microscope objective and then directed at an optical spectrum analyser (AQ-6315A, Ando Electric Co.) placed at a distance of 31 cm.
The experimental set-up is shown in Fig. 3.
Each sample, placed 15 cm from the Supercontinuum source and put between two circular steel plates having a central hole (1.5, 2.8 and 3.8 mm diameter), was held without pressure to avoid any change in its size. (Fig. 4)
The different thickness of each sample.
Tissue | Thickness | |
---|---|---|
1 | Nerve | 0,2 mm. |
2 | Skin | 0,3 mm. |
3 | Small Intestine | 0,1 mm. |
4 | Lung | 0,3 mm. |
5 | Esophagus | 0,4 mm. |
6 | Stomach | 0,8 mm. |
7 | Kidney | 2,4 mm. |
8 | Heart | 2,2 mm. |
9 | Spleen | 1,2 mm. |
10 | Liver 1 | 5,7 mm. |
11 | Liver 2 | 2,3 mm. |
12 | Liver 3 | 0,6 mm. |
13 | Muscle 1 | 2,5 mm. |
14 | Muscle 2 | 0,3 mm. |
15 | Bone | 2,2 mm. |
The analyser registered the intensity values ranging from 350 nm to 1700 nm with a resolution bandwidth of 10 nm and reported in the screen the resulting graph with wavelengths (in nm) on the abscissa and power (in dBm) on the ordinate. (Fig. 5)
The experimental set-up used for the measurements.
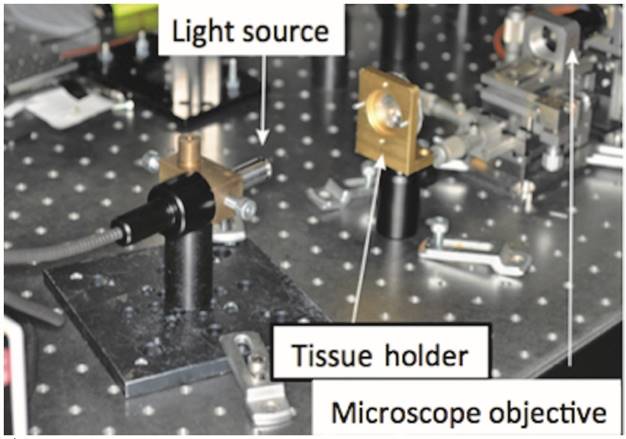
The couples of the plates and the support used for the test.
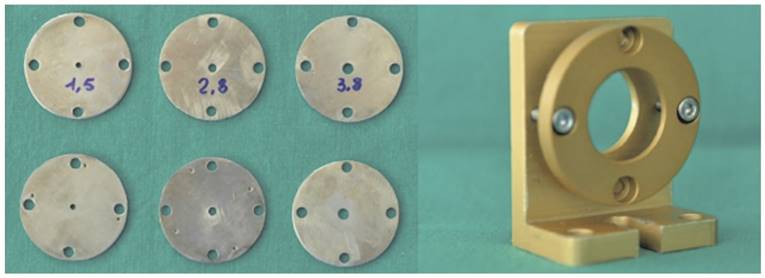
The graph obtained by the analysis: device screen (left) and graphic by Excel (right).
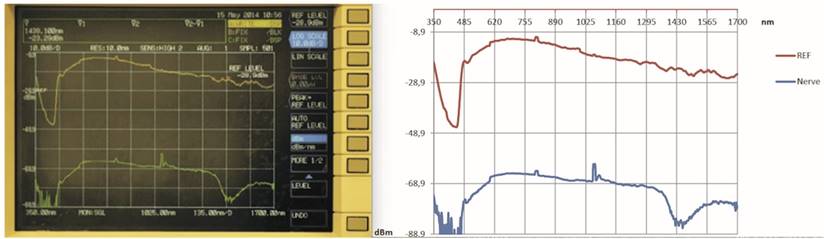
Results and discussion
In Fig. 6 and Fig. 7, the graphs obtained for stomach and kidney samples are shown with wavelengths (in nm) in the horizontal axe and energy transmitted (in dBm) in the vertical axe; dBm is the ratio between the emitted power and 1 mW, while the energy peak around 1000 nm wavelength and observed in all the graphs is generated by the supercontinuum pump (1064 nm).
In Table 2 and Fig. 8, the different transmission energies in the samples are indicated in relationship with some of the most popular wavelengths used in LLLT and surgery.
Intervals of transmitted wavelengths for each of the tested samples.
Tissues | Interval 1 (nm) | Interval 2 (nm) |
---|---|---|
Nerve | 350-475 | 1403-1519 |
Skin | 350-594 | 1149-1700 |
Small intestine | 350-476 | 1412-1490 |
Lung | 350-637 | 1359-1700 |
Oesophagus | 350-588 | 1390-1577 |
Stomach | 350-667 | 1177-1390 |
Kidney | 350-615 | 1396-1524 |
Heart | 350-629 | 1376-1700 |
Spleen | 350-752 | 1351-1700 |
Liver 1 (5.7) | 350-1057 | 1070-1700 |
Liver 2 (2.3) | 350-667 | 1323-1700 |
Liver 3 (1.5) | 350-617 | 1381-1700 |
Muscle 1 (1.5) | 350-590 | 1388-1600 |
Muscle 2 (0.3) | 350-602 | 1328-1700 |
Bone | 350-599 | 1388-1700 |
The tests demonstrated that all the processed samples have different behaviours in relationship with the wavelengths used.
In Fig. 8 the ranges of the wavelengths transmitted through each sample are reported and evidenced the most commonly used lasers in surgery. This underlines that most of them do not seem to be the proper approach.
Biological tissues may be considered such media which have a higher average refractive index than air [25] and characteristic optical parameters of absorption; the propagation of the light inside them is in function both of the scattering and absorption coefficients of its elements, i.e. cells, subcellular components and different structures [26].
As reiterated in the Introduction, for a monochromatic light, such as a laser, the optic absorption coefficient for each homogeneous and isotropic sample can be calculated with the Beer-Lambert law [27]; nevertheless, the quantity of absorption due to the light scattering must also be considered.
The main parameters related to the propagation of the light in the tissues are the density, the size and the shape of the sample, as well as the polarization of the incident light and the refractive index [28].
The knowledge of these effects and, particularly, the identification of the most absorbed wavelengths in a tissue is fundamental in clinical applications, considering the great number of lasers on the market today and the growing number of laser treatments suggested in the medical field.
Due to the structure variability in the different kinds of biological tissue, various methods have been employed to analyse the optic absorption; the two main methods consider tissue a medium with a random distribution of continuous optic parameters or such a group of scattering elements, and the choice of the method depends on both the studied samples' structural specificity and the light-scattering characteristics [29].
The “Monte Carlo (MC)” approach, used in different disciplines, consists in the numerical simulation of photon transport inside media and has also been applied to study scattering in tissue [30, 31].
An interesting work, by Lomelì-Mejia et al [32], analysed the behaviour of different wavelengths on the skin of rats and rabbits using the Photoacoustic Spectroscopy (PAS): the advantage was its utility for transparent, opaque, and highly scattering tissues and no need for particular treatments or reagents.
Results demonstrated a relationship between absorption and roughness, melanin quantity and density of the processed tissue and that it is inversely proportional to the wavelength.
On the contrary, this study, utilizing a supercontinuum white light laser, may be performed by many wavelengths on the same tissue to compare, at the same time, all the wavelengths ranging from 350 to 1700 nm.
Results showed that, generally, each irradiated tissue had no transmission in two wavelength intervals, the first one (350-600 nm) in the visible section of the “therapeutic window” due to the presence of chromophores, the other one in the infra-red portion (1300-1700) due to the water absorption peak.
Regarding the visible spectrum, the non-transmitted wavelengths in all the samples are in the blue and green, suggesting the utilisation of 450 nm diode and potassium-titanyl-phosphate lasers in LLLT and surgery [33, 34].
In the infra-red, the use of some wavelengths that are considered very effective in biostimulation and surgery, i.e. 810 nm and 980 nm diodes and Nd:YAG, do not seem advisable while the employment of devices emitting in far infra-red (Nd:YAP and 1470 nm diodes) with a good absorption in the water is justified. [35, 8].
Graph obtained by stomach sample.
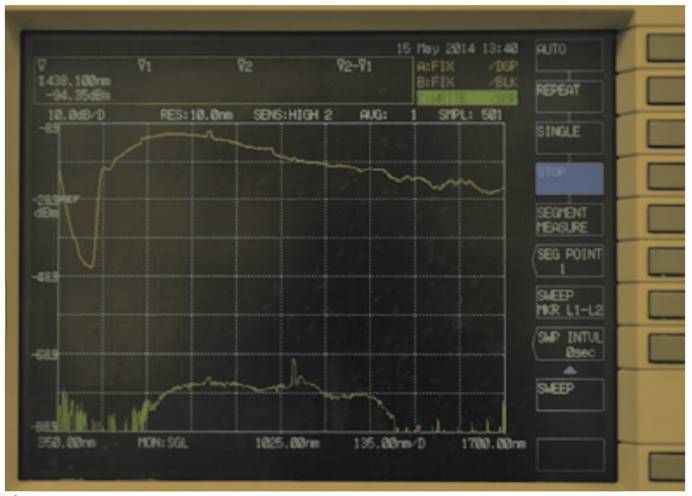
Graph obtained by kidney sample.
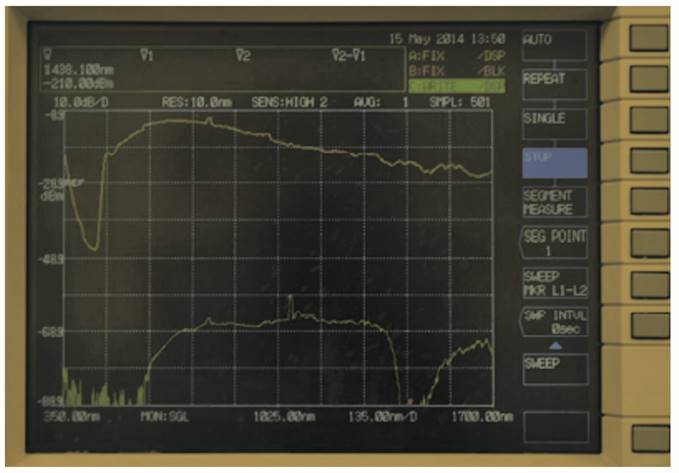
Graphic visualization of the wavelengths transmitted by the different samples in relationship with the most commonly used laser in surgery and LLLT (in increasing order: Blue diode, KTP, red diode, IR diode, Nd:YAG, Nd:YAP and IR diode).
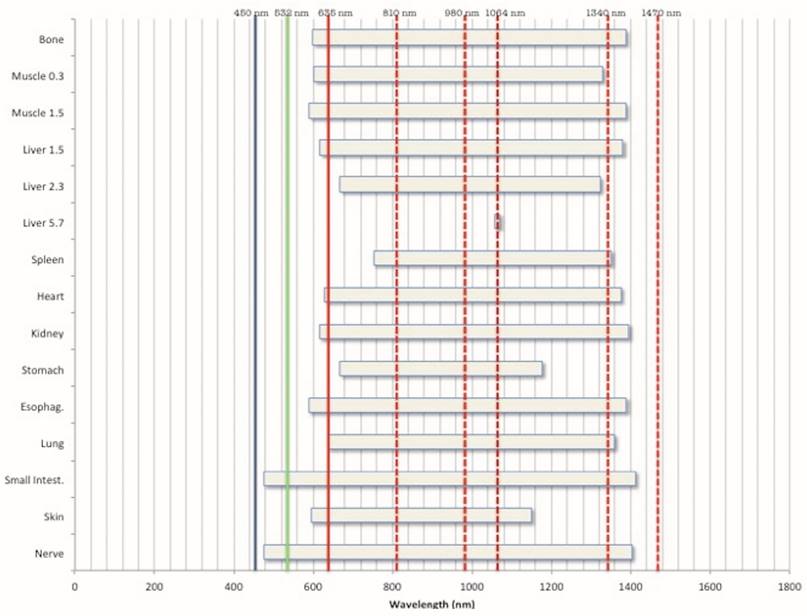
Conclusions
Even if an “ex vivo” study, due to the different conditions of the processed tissues if compared to the living organs (water contains, blood circulation, etc.), the use of the Supercontinuum device seems to be a good way for investigating the laser-tissue interactions in different biological tissues, and it may suggest important indications in the field of biostimulation and surgery.
The results obtained new opportunities on the clinical utilisation of wavelengths emitting in the blue and green and propose new indications for infra-red lasers, by favouring the wavelengths emitting beyond 1000 nm and showing that the use of ranges around 800-1000 nm, which are at the moment the most popular in surgery, is not very advantageous in terms of laser-tissue interactions.
Acknowledgements
The authors acknowledge Prof. Paolo Vescovi and his colleagues at the University of Parma for the DONATION of study samples obtained by their study published in Supportive Care Cancer [Mergoni G, Vescovi P, Sala R, Merigo E, Passerini P, Maestri R, Corradi D, Govoni P, Nammour S, Bianchi MG. The effect of laser therapy on the expression of Osteocalcin and Osteopontin after tooth extraction in rats treated with zoledronate and dexamethasone. Support Care Cancer. 2016 Feb;24(2):807-13].
Competing Interests
The authors have declared that no competing interest exists.
References
1. Siân Harris. Lasers in Medicine. Bellingham WA, USA: SPIE Professional. 2011
2. Law KS, Abbott JA, Lyons SD. Energy sources for gynaecologic laparoscopic surgery: a review of the literature. Obstet Gynecol Surv. 2014;69(12):763-76
3. Fornaini C. Er:YAG and adhesion in conservative dentistry: clinical overview. Laser Ther. 2013;22(1):31-5
4. Kasner SE, Rose DZ, Skokan A et Al. NEST-2 Steering Committee and Investigators. Transcranial laser therapy and infarct volume. Stroke. 2013;44(7):2025-7
5. Mester A. Laser biostimulation. Photomed Laser Surg. 2013;31(6):237-9
6. Ackroyd R, Kelty C, Brown N, Reed M. The history of photo-detection and photodynamic therapy. Photochem Photobiol. 2001;74(5):656-69
7. Merigo E, Clini F, Fornaini C, Oppici A et Al. Laser-assisted surgery with different wavelengths: a preliminary ex vivo study on thermal increase and histological evaluation. Lasers Med Sci. 2013;28(2):497-504
8. Fornaini C, Merigo E, Sozzi M et Al. Four different diode lasers comparison on soft tissues surgery: a preliminary ex vivo study. Laser Ther. 2016;25(2):105-114
9. Anders JJ, Moges H, Wu X, Erbele ID et Al. In vitro and in vivo optimization of infrared laser treatment for injured peripheral nerves. Lasers Surg Med. 2014;46(1):34-45
10. de Brito Vieira WH, Bezerra RM et Al. Use of low-level laser therapy to muscle fatigue resistance: a randomized double-blind crossover trial. Photomed Laser Surg. 2014;32(12):678-85
11. Macias DM, Coughlin MJ, Zang K et Al. Low-Level Laser Therapy at 635 nm for Treatment of Chronic Plantar Fasciitis: A Placebo-Controlled, Randomized Study. J Foot Ankle Surg. 2015 Mar 10
12. Kushibiki T, Tajiri T, Ninomiya Y, Awazu K. Chondrogenic mRNA expression in prechondrogenic cells after blue laser irradiation. J Photochem Photobiol B. 2010;98(3):211-5
13. Parker S. Verifiable CPD paper: laser-tissue interaction. Br Dent J. 2007;202(2):73-81
14. Welch AJ, Torres JH, Cheong WF. Laser physics and laser-tissue interaction. Tex Heart Inst. 1989;16(3):141-9
15. McKenzie AL. Physics of thermal processes in laser-tissue interaction. Phys Med Biol. 1990;35(9):1175-209
16. Niemz MH. Laser-Tissue Interactions: Fundamentals and Applications. New York, USA: Springer. 1985
17. Sliney DH. Laser-tissue interactions. Clin Chest Med. 1985;6(2):203-8
18. Sinclair GN, Bao X, Elliott DS, Hamilton MW. Effect of random-telegraph laser phase on two-photon absorption. Phys Rev A. 1995;51(6):4809-4817
19. Polanyi TG. Physics of the surgical laser. Int Adv Surg Oncol. 1978;1:205-15
20. van Gemert MC, Welch AJ. Clinical use of laser-tissue interactions. IEEE Eng Med Biol. 1989;8(4):10-3
21. Borovoy M. Physics of laser beam interactions with living tissue. Clin Podiatr Med Surg. 1992;9(3):531-7
22. Alfano RR. The Supercontinuum Laser Source: Fundamentals with Updated References. New York, USA: Springer. 2005
23. Gmachl C, Sivco DL, Colombelli R, Capasso F, Cho AY. Ultra-broadband semiconductor laser. Nature. 2002;415:883-887
24. Dudley J, Genty G, Coen S. Supercontinuum generation in photonic crystal fiber. Rev Mod Phys. 2006;78:1135
25. Tuchin V. Tissue Optics. Washington, USA: SPIE Press. 2015
26. Minet O, Muller G, Beutan J. Selected Papers on Optical Tomography, Fundamentals and Applications in Medicine. Washington, USA: SPIE Press. 1998
27. Sassaroli A, Fantini F. Comment on the modified Beer-Lambert law for scattering media. Phys Med Biol. 2004;49:N1-N3
28. Vo-Dinh T. Biomedical Photonics Handbook. Boca Raton, Florida, USA: CRC Press. 2014
29. Schmitt JM, Kumar G. Turbulent nature of refractive-index variations in biological tissue”. Opt Lett. 1996;21:1310-1312
30. Soboll I M. A Primer for the Monte Carlo Method. Boca Raton, Florida, USA: CRC Press. 1994
31. Wilson BC, Adam GA. Monte Carlo model for the absorption and flux distribution of light in tissue. Med Phys. 1983;10:824-830
32. Lomelì-Mejia P, Alanis-Carbajal J, Cruz-Orea A, Jimenez-Perez JL. Optical Absorption coefficients of biological tissues obtained by photoacoustic spectroscopy. Rev Mex Ing Biom. 2009;1:13-17
33. Fornaini C, Rocca J-P, Merigo E. 450 nm diode laser: A new help in oral surgery. World J Clin Cases. 2016;4(9):253-257
34. Fornaini C, Rocca JP, Merigo E et Al. Low energy KTP laser in oral soft tissue surgery: A 52 patients clinical study. Med Oral Patol Oral Cir Bucal. 2012;17(2):287-91
35. De Santis D, Bertossi D, Zanotti G et Al. Nd:YAP laser assisted frenulectomy: a case series on 23 patients. Minerva Stomatol. 2013 Aug 1
Author contact
Corresponding author: Dr. Carlo Fornaini, carloit.
Received 2016-8-1
Accepted 2016-10-10
Published 2017-1-1