J Biomed 2018; 3:40-49. doi:10.7150/jbm.23390 This volume Cite
Review
Muscle and Bone Mass Loss in the Elderly Population: Advances in diagnosis and treatment
1. Department of Education, Physical Education and Health Programs, San Juan, PR, USA
2. Research and Development Service (151), VA Caribbean Healthcare System, San Juan PR, USA
3. Department of Biology, University of Puerto Rico, Mayaguez Campus, Mayaguez, PR, USA
4. Department of Biology, University of Puerto Rico, Rio Piedras Campus, San Juan, PR, USA
5. Department of Radiological Sciences, University of Puerto Rico, Medical Sciences Campus, San Juan, PR, USA
Abstract
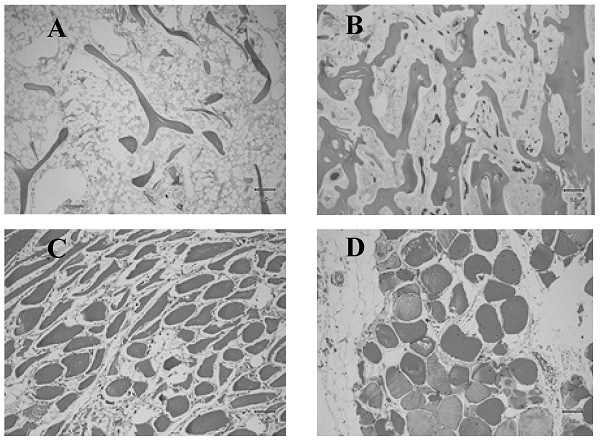
Aging is the result of different functional changes leading to a substantial reduction of all human capabilities. A variety of anatomical and physiological changes occur with advancing age. These changes are more evident in the elderly population. There are various methods to measure muscle and bone mass loss, but the dual X-ray absorptiometry (DXA) is considered one of the most efficient. The elderly population (65 years and older) has been increasing throughout the years. Loss of muscle mass (sarcopenia) and loss bone mass (osteopenia or osteoporosis) with advancing age, when untreated, represent a major public health problem for the elderly population and may result in loss of independence in later life. Untreated age-related sarcopenia and osteopenia/osteoporosis increase the risk for falls and fractures, making older individuals more susceptible to the development of mobility limitations or severe disabilities that ultimately affect their capacity for independence. In this review, we will discuss the muscle and bone mass loss in the elderly population and advances in diagnosis and treatment.
Keywords: Sarcopenia, Osteopenia, Osteoporosis
Muscle and Bone Mass Loss Measured by Dual X-ray absorptiometry (DXA) in the Elderly Population
Human aging is an irreversible and inexorable process characterized by morphological, functional and biochemical changes in the human body, including the musculoskeletal system [1]. This system gradually changes and acquires specific structural and morphological characteristics, which mainly include loss of muscle mass, strength and bone mass [2]. Loss of muscle mass and function (sarcopenia) and loss bone mass (osteopenia or osteoporosis) with advancing age, when untreated, represent a major public health problem for the elderly population and may result in loss of independence in later life. Untreated age-related sarcopenia and osteopenia/ osteoporosis increase the risk for falls and fractures, making older individuals more susceptible to the development of mobility limitations or severe disabilities that ultimately affect their capacity for independence [3].
The elderly population (65 years and older) has been increasing throughout the years. In 2010, an estimated 524 million people were aged 65 years or older globally, representing 8% of the world's population [4]. By 2050, this number is expected to nearly triple to about 1.5 billion individuals aged 65 years or older [4]. The world's population percentage for people over 65 years will double from about 11% to 22%, consequently, by 2050 there will be 2 billion people aged 65 or older living worldwide. Of those, approximately 400 million will be 80 years or older [5, 6]. With an increase in the elderly, comes an increase in diseases and conditions that commonly affect this population.
Physiological and Anatomical Changes Related to Aging
Changes | Functional Effects | |
---|---|---|
Cardiovascular | - Increased collagen matrix in Tunica Media - Loss of elastin fibers - Cardiac hypertrophy: septum thickening - Decreased cardiomyocytes and increased extracellular matrix | - Heart and vascular stiffness - Mayor endothelial dysfunction - Explosive volume preserved - Increased risk of arrhythmias |
Renal | - Thinning renal cortex - Glomerular sclerosis arteries - Glomerular basement membrane Thickening | - Decreased ability to concentrate urine. Lower renin and aldosterone levels - Lower vitamin D hydroxylation |
Glucose Metabolism | - Increased visceral fat - Fat tissue infiltration - Less beta cell mass | - Increased production of adipokines and inflammatory factors inflammatory factors - Greater insulin resistance and diabetes |
Bones | - Decreased bone mineral content | - Increased fractures and falls - Osteoporosis |
Muscular | - Loss of muscle mass - Less type II fibers - Fat infiltration | - Decreased strength and power - Falls - Fragility |
Central Nervous System | - Less brain mass - Increased cerebrospinal fluid - Low neuronal loss, focused - Changes in neuronal arborization | - Less targeting neuronal activity - Lower processing speed - Decreased working memory - Less motor skills |
Body Composition | - Increased body fat - Increased Body Mass Index (BMI) | - Increased risk of disease. |
Aging causes a variety of anatomical and physiological changes (Table 1). Knowledge of age-related anatomical and physiological changes allows for the understanding of the pathophysiological differences between the elderly and the rest of the adult population. Muscle mass loss is one of the multiple age-related physical changes involving a condition known as sarcopenia [7], which has been associated to the loss of physical activity [8]. Muscle mass loss is thought be related to motor neuron loss due to the aging process [9]. Another disorder related to aging is bone mass loss, known as osteopenia, which can further progress to osteoporosis [10]. Both conditions are multifactorial, but genetics, nutrition, and life style have been shown to be associated with these disorders [10]. The extent of loss in both of these conditions can be measured with a technique called Dual energy x-ray absorptiometry (DXA). The gold standard for measuring bone material properties in clinical practice is axial DXA measurement from femur and spine [10]. There are multiple treatments for both of these conditions including the use hormones and a balanced nutrition; however, exercise is the most recommended therapeutic approach for both disorders [10]. This article intends to compile actualized information about muscle and bone mass loss in the elderly population.
Muscle Mass Loss
Muscle mass loss is an age-related condition and one of the physiologic changes involved in sarcopenia. The European Working Group on Sarcopenia in Older People (EWGSOP) defines sarcopenia as a progressive and generalized loss of skeletal muscle mass and strength, or physical performance [11]. Muscle mass loss has a cause-effect relationship with muscle strength. Loss of both muscle mass and strength, increase with age and are a problem for the elderly since it can result in a poor quality of life and physical disabilities [11].
Muscle mass loss can be caused by various factors, including: disease, decreased caloric intake, poor blood flow to the muscle, mitochondrial dysfunction, a decline in anabolic hormones, and an increase in pro-inflammatory cytokines [8]. However, disuse coupled with aging are the major underlying causes [8]. Aging is associated with an increase in mitochondrial abnormalities, which lead to damages in mitochondrial membrane permeability, pores and result in apoptosis [8, 12]. Another factor related to aging and sarcopenia is poor blood flow to muscle capillaries due to a decline in nitric oxide production [8, 13]. Weight loss is also associated with the development of muscle mass loss. In addition, studies have shown that aging is associated with a physiological anorexia that leads to weight loss [13, 14], and weight loss results in a 75% loss of fat and a 2% loss of muscle and bone [8]. Age-related loss of motor neurons is a major component of sarcopenia and muscle mass loss [9], which results in a decrease in muscle function caused by disuse. The Motor Unit Number Index (MUNIX) is a neurophysiological measure that provides an index of the number of lower motor neurons in a muscle using the compound muscle action and surface electromyographic interference pattern [15]. Weaker individuals exhibit a smaller motor unit number index (MUNIX) value [9, 16]. The loss of functioning motor units may be partially responsible for weakness often seen in the elderly [16]. Loss of anabolic hormones, such as testosterone, DHEA, growth hormone, and insulin-growth factor 1, occurs with aging [8]. It has been shown that testosterone regulates muscle mass and strength [17, 18]. Other factors such as satellite cells have an essential role in muscle repair and growth, and are highly influenced by its surrounding physiological milieu and very sensitive to oxidative stress, which is elevated in aged muscles [19]. Insulin resistance occurs with aging and is associated with obesity [8]. Insulin resistance plays an important role in decreasing available glucose and protein for muscle anabolism [20]. Moreover, obesity and concomitant diseases lead to an increase in pro-inflammatory cytokines such as interleukin-6 (IL-6), interleukin-1 (IL-1), and tumor necrosis factor alpha (TNF- α) [8]. People with diabetes mellitus have accelerated muscle loss [21, 22]. Stroke and hip fracture are highly prevalent among the elderly and usually rapidly lead to an increase in muscle loss [23, 24]. This appears to be due to disuse and inflammation, stroke, and/or denervation.
Prevalence of Muscle Mass Loss
The prevalence of muscle mass loss is increasing and is expected to continue to rise in the years to come. Five to thirteen percent of older persons of 65 years and older have low muscle mass; the percentage increases up to 50% in persons that are over 80 years old [17, 25]. By the age of 80, it is estimated that 40% of the muscle mass present at age 20 is lost [26]. In a study with 198 subjects, 25% of the patients in a geriatric ward were considered to be sarcopenic [27]. The increased prevalence of sarcopenia is not unique to the United States. In Italy, about 20% of community-dwelling persons were reported to have low muscle mass [28]. In Barcelona, Spain, low lean muscle mass was present in 33% of elderly women and 10% of elderly men [29]. According to Wu et al., low muscle mass was present in 2.5% of community-dwelling women and 5.4% of men in Taiwan [30]. Currently, sarcopenia affects more than 50 million people worldwide and is expected to affect 200 million individuals in the next 40 years.
Bone Mass Loss
Bone mass loss is a condition known as osteopenia. Osteopenia often progresses to osteoporosis, a condition characterized by the reduced bone mineral density and increased rate of bone loss [10]. Bone mineral density decreases with age. Therefore, the probability of a person suffering from osteopenia or osteoporosis, and related skeletal fragility increases with age [10].
The causes leading to bone mass loss are multifactorial and similar to the causes of muscle mass loss. The most common cause of osteopenia is aging. Skeletal aging is known to progress faster in women than in men due to hormonal changes after menopause [10]. However, genetics, nutritional factors, lifestyle factors, and comorbidities have been shown to be associated with bone mass loss [10, 31]. During the later years of an individual's lifespan, there is an accelerated loss of bone tissue, which is probably related to genetic factors [32, 33]. There are multiple genes involved in the control of musculoskeletal interactions [34, 35, 36, 37], such as the alpha actinin-3 (ACTN3) and the myocyte enhancer factor 2C (MEF2C) gene. Alpha Actinin-3 regulates the muscular power performance and it is associated to decreased bone mass, whereas Myocyte Enhancer Factor 2C is responsible for controlling bone development by means of activating chondrocyte hypertrophy [37]. With “normal” aging, there is a marked increase in the formation of advanced glycation end-products (AGEs) [37]. In bones, AGEs enhance osteoclast-induced bone resorption, modify bone proteins and disturb bone remodeling [37, 38]. In addition to genetics, the whole process of maturation, development, and decline of the musculoskeletal system is significantly affected by environmental influences [39]. An example of an environmental factor would be a fall, which can increase the possibility of developing osteopenia and osteoporosis [39]. In females, at the beginning of menopause, the acute loss of the restraining effect of estrogen on osteoblasts and osteoclasts membrane receptors leads to accelerated bone turnover and the uncoupling bone formation from resorption [40]. The imbalance between calcium secretion and absorption following the estrogen depletion has been suggested to influence the accelerated bone loss rate in women [10]. Calcium and vitamin D deficiency may increase the possibility of suffering from accelerated bone mass loss. High alcohol intake and smoking can also contribute to bone loss [41]. In addition, high alcohol intake is associated with increased bone loss, falling, and fractures in older men [42]. Smoking increases the risk of a fracture and osteoporosis [41].
Prevalence of Bone Mass Loss
In 2010, it was estimated that more than 99 million adults aged 50 years and older had severely decreased bone density mass in the United States [43]. Based on the overall 10.3% prevalence of osteoporosis, it was estimated that in 2010, 10.2 million older adults (65 years and older) had osteoporosis in the United States. The overall prevalence of low bone mass was 43.9%, from which it was estimated that 43.4 million older adults had osteopenia, from mild to severe levels [43]. It is projected that by 2020, the number of adults over age 50 with low bone mass, including osteoporosis, will grow from approximately 54 million to 64.4 million. By 2030, that number will further increase to 71.2 million (a 29% increase from 2010) [44]. It is anticipated that the number of fractures will grow proportionally [44].
Muscle and Bone Mass Measurement Methods
There are various methods to measure sarcopenia and osteopenia, but dual X-ray absorptiometry (DXA) is considered the most accurate. Muscle mass can be assessed in an accurate manner by dual X-ray absorptiometry (DXA) with a very low dose of radiation (1-2 micro-Sieverts) [12]. However, there are still challenges to overcome using this method to consistently diagnose sarcopenia and osteopenia or osteoporosis.
Histologic images of normal and anomalous bone and muscle. A) Femoral head showing delicate bone trabeculae suggestive of osteoporosis. B) Femoral head showing relatively normal bone trabeculae. C) Atrophied muscle. D) Normal muscle.
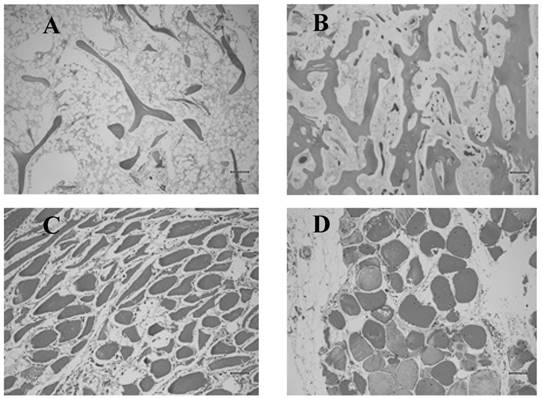
Despite advances on the field of musculoskeletal physiology, low muscle mass and related sarcopenia remain inconsistently identified due to a lack of standardized diagnostic approaches and the varying definition of normal lean mass. It has been recently suggested that normal muscle mass is a reflection of a variable combination of body composition indices and cutoffs, that at the same time are influenced by genetics and environmental factors. Among the basic determinants of a normal lean mass are gender, age, fat mass, height, ethnicity, body region, hydration status, and interactions with medications. According to Bosy-Westphal et al., the definition of lean mass depletion should be based on an indirect or direct measure of skeletal muscle mass, normalized for height and skeletal muscle index, in combination with fat mass [45]. Fat Free Mass (FFM) is defined as the sum of all non-lipid components of the body and is often normalized for body size [45]. FFM is either expressed as a percentage of body weight (% FFM) or by dividing FFM by height squared (fat-free mass index (FFMI) = FFM (kg) per height (m2)). The Skeletal Muscle Index (SMI) uses the combination of the lean (or fat-free) soft tissue mass of the four limbs from a DXA scan, known as appendicular skeletal muscle mass (ASM). SMI is defined as appendicular skeletal muscle mass/height2 (kg/m2) [17]. However, both indices have limitations with increasing age or increasing body fat mass. Janssen et al., proposed a skeletal muscle mass index adjusted for weight (ASM/weight) that significantly correlated to functional impairment and disability [46]. In 2014, the Foundation for the National Institutes of Health (FNIH) Sarcopenia Project recommended a new muscle mass measurement normalized to the body mass index (BMI), the ASM/ BMI index [42, 47, 48]. The use of the ASM/BMI index has been rapidly increasing. The most recent reference values for FFMI, and appendicular SMI are gender, age and ethnicity specific and were published for different percentiles applicable to individuals 8 to 85 years old. The values were derived from the National Health and Nutrition Examination Survey (NHANES) population-based sample acquired with modern DXA fan beam scanners in 15 counties across the United States from 1999 through 2004 [49].
In 1994, the World Health Organization (WHO) provided an operational definition of bone mass loss using a standardized score, called T-score, to describe the categories of bone loss by comparing Bone Mass Density (BMD) to average values in young healthy women. T-score indicate the magnitude of the standard deviation from the normal in terms of bone mass density. The categories for diagnosis were defined as: normal (T-score -1.0 and above), low bone mass, referred to as osteopenia (T-score between -1.0 and -2.5), osteoporosis (T-score -2.5 and below), and severe osteoporosis (T-score -2.5 and below with history of a fracture). These criteria applied only to white postmenopausal women since the research data was primarily limited to this group. In addition, diagnosis can only be based on three skeletal sites of measurement: lumbar spine, hip, or forearm. The NIH Consensus Development Panel on Osteoporosis defined osteoporosis as a skeletal disorder characterized by compromised bone strength that predisposes a person to an increased risk of fracture. More recently, in 2008, an after the second global meeting in 2004 that promoted extensive scientific debate, the revised WHO definition for osteoporosis was released. This new assessment includes bone mass density with selected risk factors for fracture in addition to height and weight. A fracture risk score, called FRAX, is calculated to determine your 10-year probability of fracture. Two scores are given: probability of hip fracture and major osteoporotic fractures (defined as wrist, shoulder, hip, or painful spine fractures).
In 2015 the International Society for Clinical Densitometry (ISCD) updated the new measurements used to diagnose patients with loss of bone mass. Two of these introduced measurements, known as “Trabecular Bone Score (TBS)” and “Hip Geometry”, are rapidly gaining use in clinical practice. TBS is associated osteoporotic and hip fracture risk in postmenopausal women and men over the age of 50. An advantage of TBS is that it can be used in association with FRAX and BMD to adjust FRAX probability of fracture in postmenopausal women and older men. However, it is important to mention that TBS is not used alone to determine treatment recommendation in clinical practice and it is not useful for monitoring bisphosphonate treatment in postmenopausal women. Hip geometry uses mostly the hip axis length parameter (HAL), which is derived from DXA, because of its association with hip fracture risk in postmenopausal women. However, the ISCD recommends that HAL and other parameters derived from DXA (CSA, OD, SM, BR, CSMI and NSA), should not be used to initiate treatment or for monitoring [50].
Treatment of Muscle Mass Loss
Testosterone
In the elderly, the testosterone concentrations decrease with age. Lower testosterone concentrations are associated to lower fat-free mass, lower appendicular skeletal muscle mass, and lower force on the knee extension in hypogonadal men versus healthy controls [51]. The prevalence of hypogonadism is 20% in men over 60 years and up to 50% in men over 80 years. In a controlled, double-blinded, randomized clinical trial by Wittert et al., the administration of 80 mg of oral testosterone every 12 hours for 12 months, increased lean body mass by 2% while fat mass decreased in 69 hypogonadal subjects [52]. Testosterone increases muscle protein synthesis, but its effects on muscle are modulated by several factors, including genetic, nutritional, and exercise history [53].
Estrogen
It has been suggested that the transition to menopause, and the subsequent decrease in estrogen, may play a role in the loss of muscle mass [54]. According to Van Geel et al., there is a positive correlation between lean body mass and estrogen levels [55]. Additionally, estrogen may have a direct effect on muscle mass, as the cell membranes of skeletal muscle contain beta estrogen receptors [56]. The mechanisms by which the decrease in estrogen levels may have a negative effect on muscle mass remains not well understood. However, it may be associated with increased pro-inflammatory cytokines such as TNF- α and IL-6, which could be involved in the development of sarcopenia [57]. In younger women (mean 55 years old), estrogen and progestin replacement therapy (three HRT cycles, Trisequens Forte, Novo Nordisk, Bagsvaerd, Denmark) of 17 bestra-diol (4 mg for 22 days and 1 mg for 6 days) and cyclicnorethisterone acetate (1 mg for 10 days of each 28-daycycle) has proven effective in increasing lean mass and decrease body fat mass after 6 months of treatment [58].
Human growth hormone (HGH)
Treatment with HGH is very effective in promoting bone and muscle growth, and has been approved by the FDA for a number of indications [59]. HGH replacement therapy increases muscle mass and strength in young adults with hypopituitarism [60]. In middle age, HGH has an anabolic effect. In adults over 50 years with HGH deficiency that started in adulthood, treatment increases the strength of the thigh in both sexes [61].
Nutrition
Reducing food intake in the elderly has consequences that could be important for muscle mass and strength. Reduction of energy intake corresponding to lower levels of energy consumption, leads to weight loss and ultimately, to muscle mass loss [62]. A number of interventions have been studied, ranging from the provision of nutritional support [63] to supplementation with specific nutrients [64, 65]. The nutrients that have been most consistently linked to sarcopenia and frailty in the elderly are vitamin D, protein, and a series of antioxidants and nutrients, including carotenoids, selenium, and vitamins E and C [66].
Proteins
Proteins are considered a key nutrient in the elderly [67]. They provide the necessary energy source for muscle protein production as absorbed amino acids have a stimulating effect on muscle protein synthesis after feeding [68]. There is evidence that the synthetic response to amino acid intake can be mitigated in the elderly, particularly when consumption is low [67] and when protein is consumed with carbohydrates [69]. Protein intake should be carefully increased in the elderly to maintain nitrogen balance and protection against muscle loss [67]. Protein and amino acid supplements have the potential to slow down the development of sarcopenia [70].
Strength training
One of the most effective ways to combat the loss of muscle mass is by stimulating hypertrophy and increasing muscle strength by incorporating strength training [71]. Strasser et al., showed that after 6 months of strength training 3 times per week, maximum lean body mass increased by 1.0 ± 0.5 kg in older adults [72]. In a randomized controlled trial, Binder et al., studied the effects of strength training on 91 subjects with community residents' fragility syndrome (78 years old and over) [73]. Three months of supervised strength training caused an increase in fat-free mass in the thigh muscle and the whole body in older women and men [73]. In a study involving elderly men with different types of training approaches, it was shown that strength training maintained muscle mass and performance more efficiently than other types of exercise [74].
Treatments for Bone Mass Loss
Adequate Nutrition
Changes in lifestyles, such as having an adequate diet, can exert a profound effect on the progression of osteopenia. For example, increasing the intake of calcium and vitamin D to the daily recommended levels can promote musculoskeletal health [41]. The National Osteoporosis Foundation (NOF) recommends adults under age 50 to have an intake of 1,000 mg of calcium and 400-800 IU of vitamin D daily and adults age 50 and older to have and intake of 1,200 mg of calcium and 800-1,000 IU of vitamin D daily. Products that are high in calcium are low-fat and non-fat milk, yogurt, and cheese [75]. Vitamin D can be obtained through careful exposure to sunlight, dietary supplements, and food nutrients [75]. After revision of meta-analyses and clinical trials of older community-dwelling and institutionalized persons, the workgroup concluded that a serum 25 hydroxyvitamin D (25(OH) concentration of 30 ng/mL (75 nmol/L) should be the minimum goal to achieve in older adults, particularly in frail adults, who are at higher risk of falls, injuries, and fractures [76].
Exercise
Specifically weight training and walking are beneficial for increasing bone density in middle-aged and older people [77]. Regular weight-bearing and muscle-strengthening exercises can reduce the risk of falls and fractures [78, 79, 80, and 81]. This type of exercise can increase the bone density as well as the strength by micro-architectural bone arrangement. A 6-month Tai Chi program was shown to be effective in decreasing the number of falls, the risk of falling, and the fear of falling, in addition to improving functional balance and physical performance in physically inactive persons aged 70 years or older [82]. Also, most physically active individuals have the lowest serum sclerostin levels [83].
Reduce alcohol and tobacco consumption
Consuming high quantities of alcohol and smoking are detrimental to musculoskeletal health. Excessive alcohol intake is considered detrimental for bone health [84]. More than two drinks per day for women and more than three drinks per day for men have been shown to increase the risk of falling [84]. The use of tobacco products is damaging to musculoskeletal health. Orthopedic perioperative complications of smoking include impaired healing, increased infection, delayed and/or impaired fracture union and arthrodesis, and inferior arthroplasty outcomes [85]. The National Osteoporosis Foundation recommends that people participate of tobacco cessation programs [78].
Medications
Different countries have established different criteria regarding when osteoporosis medicine is recommended. In the United States, the National Osteoporosis Foundation (NOF) released new guidelines in 2008. Treatment guidelines were established based on fracture risk and a cost-effective model for lowering risk with the use of osteoporosis medicines. The guidelines for treatment are for postmenopausal women of any race or ethnicity and men age 50 years and older. The three major categories considered as high-risk groups for the development of fractures and that would benefit from FDA approved treatment options are: people with history of fracture of the hip or spine, individuals with BMD in the osteoporosis range (T-score of -2.5 or lower), and BMD in the low bone mass or osteopenia range with a higher risk of fracture defined by FRAX score for major osteoporotic fracture 10-year probability of 20% or higher OR hip fracture 10-year probability 3% or higher [41, 86].
The major mechanisms related to therapeutic approaches for bone mass loss are antiresorptive drugs and anabolic drugs [77]. Antiresorptive agents decrease bone loss and increase bone strength [84]. The anabolic agents are recombinant proteins that stimulate positive bone formation balance and subsequent bone remodeling [87]. The categories of drugs used for the treatment of osteoporosis can be summarized as: bisphosphonates, the selective estrogen receptor modulators (SERMs), parathyroid hormone (PTH), calcitonin, hormone replacement therapy (HRT), and humanized monoclonal antibody directed against the receptor activator of the nuclear factor-kappa B ligand (RANKL).
Bisphosphonates
Alendronate sodium is approved by the FDA for the prevention and treatment of postmenopausal osteoporosis. Alendronate is also used to increase bone mass in men with osteoporosis and for the treatment of osteoporosis in men and women taking glucocorticoids [89]. Alendronate reduces the incidence of spine and hip fractures by about 50% over 3 years in patients with a prior vertebral fracture or in patients who have osteoporosis at the hip [90]. Zoledronic acid is the most potent bisphosphonate available. It increases BMD at the spine by 4.3-5.1% and the hip by 3.1-3.5%, as compared with placebo. Over 3 years, it reduces the incidence of spine fractures by 70%, hip fractures by 41%, and nonvertebral fractures by 25% [91]. Ibandronate is another bisphosphonate that can be given orally once a month [92]. Intravenous bisphosphonates (given once every three months) are excellent choices for patients intolerant of oral bisphosphonates or for those in whom adherence is an issue [92]. Other oral bisphosphonates include risedronate or risedronate delayed-release, which can be given daily, weekly, or monthly [92]. Risedronate reduced vertebral fractures by 41% and nonvertebral fractures by 39% [92].
Selective Estrogen Receptor Modulators (SERM)
Selective estrogen receptor modulators (SERMs) bind with high affinity to the estrogen receptor, and have estrogen agonist and antagonist properties that vary depending upon the individual target organ [78, 93, 94]. Some SERMs, such as raloxifene and bazedoxifene, have estrogen activity in bone and, therefore prevent bone loss, improve bone mineral density (BMD), and decrease the risk of vertebral fracture [93]. Currently, several SERMs are being utilized clinically [95]. Tamoxifen is used as breast cancer treatment, and it inhibits osteoclast-driven bone resorption [96, 97]. The SERMs raloxifene and bazedoxifene also both reportedly inhibit bone resorptive activity in post-menopausal osteoporosis patients [95, 98, 99, 100] and have been used to prevent bone fragility fractures. Binding of SERMs to estrogen receptors (ERs) modulates the receptor's conformation or ability to form a complex with co-regulators, which in turn, alters their transcriptional activity [94, 101, 102, 103, 104 and 105].
Parathyroid Hormone (PTH)
Parathyroid hormone, an 84-amino acid peptide secreted by the parathyroid gland, is an important systemic regulator of calcium homeostasis [106]. It has been demonstrated that intermittent administration of PTH leads to an anabolic effect on bone [107]. Teriparatide, a synthetic polypeptide hormone consisting of the 1-34 fragment of human parathyroid hormone, retains most of the biological activities of PTH [108]. Intermittent administration of teriparatide also has the anabolic effects that stimulate bone formation and activate bone remodeling [109], improving the microarchitecture of trabecular bone and cortical bone [110]. Previous studies reported that teriparatide also increased bone mineral density and decreased risk of vertebral and nonvertebral fractures [111, 112, 113 and 114]. Meanwhile, teriparatide was the only anabolic drug for osteoporosis adopted by the Food and Drug Administration [115].
Calcitonin
Calcitonin is a naturally occurring hormone which helps regulate calcium levels in your body and is involved in the process of bone building [116]. It can be administered by injection or nasal spray. Calcitonin treatment decreases the rate of bone thinning and relieves pain that occurs when the bones in the spine (vertebrae) break and collapse on top of each other (spinal compression fracture) [116]. It may be prescribed to women who are more than 5 years beyond menopause and who do not tolerate bisphosphonate medicines and in men with osteoporosis who have normal levels of the male sex hormone testosterone or whose osteoporosis does not get better with testosterone treatment [116].
Hormone Replacement Therapy (HRT)
The hormone replacement therapy is known to prevent accelerated bone loss [117, 118] and improve bone mass in postmenopausal osteoporosis [119]. In addition to improvement in BMD, fractures were decreased with hormone therapy [120]. In the conjugated ethinyl estradiol (CEE) medroxyprogesterone (MPA) arm of the Women's Health Initiative (WHI) study, active therapy significantly reduced fractures; however, the WHI study population consisted of women who were older than 70 years of age and who had undergone menopause more than 20 years previously [121]. This type of therapy is approved by the FDA for the prevention of osteoporosis, relief of vasomotor symptoms, and vulvovaginal atrophy associated with menopause [77]. The Woman's Health Initiative (WHI) found that 5 years of HRT with conjugated estrogens and medroxyprogesterone acetate reduced the risk of clinical vertebral fractures and hip fractures by 34% and other osteoporotic fractures by 23% [122].
Physical Medicine and Rehabilitation (PM&R)
It is known that physical medicine and rehabilitation can reduce disability, improve physical function, and lower the risk of subsequent falls in patients with osteoporosis. Moderate to vigorous physical activity is associated with a hip fracture risk reduction of 45% (95% CI, 31-56%) and 38% (95% CI, 31-44%), respectively, among men and women. Risk of falling is suggested to be generally reduced among physically active people, with a potential increased risk in the most inactive people [123]. Rehabilitation along with exercise is recognized as a means to increment musculoskeletal function, such as activities involved in daily living. Psychosocial factors also strongly affect the functional ability of patients with osteoporosis who have already suffered fractures [77]. Therefore, psychological support and therapy can improve the wellbeing and quality of life of these patients.
Conclusion
Aging causes a variety of anatomical and physiological changes. Skeletal muscle physiology is one of the most affected by aging process. Loss of muscle and bone mass results in a poor quality of life and impaired mobility. Although multifactorial, the loss of functionality and body movement acts as a major factor driving loss muscle and bone mass. The DXA measurement technique is considered the most accurate and is an effective method for measuring both, sarcopenia and osteopenia. In addition to the use of DXA as a diagnostic method, emphasis on adequate nutrition and the promotion of physical activities could help reduce the loss of muscle and bone mass among the elderly.
Disclaimer
The contents of this article do not represent the views of the U.S. Department of Veterans Affairs or the United States Government. This material is the result of work supported with resources and the use of facilities at the VA Caribbean Healthcare System in San Juan, Puerto Rico.
Acknowledgements
PRCTRC grant support - "Research reported in this publication was supported by the National Institute on Minority Health and Health Disparities (NIMHD) and the National Institute of Allergy and Infectious Diseases (NIAID) of the National Institutes of Health under Award Number U54MD007587. The content is solely the responsibility of the authors and does not necessarily represent the official views of the National Institutes of Health."
Competing Interests
The authors have declared that no competing interest exists.
References
1. Pinto CL, Botelho PB, Carneiro JA, Mota JF. Impact of creatine supplementation in combination with resistance training on lean mass in the elderly. J Cachexia Sarcopenia Muscle. 2016;7(4):413-21
2. Araújo AP, Bertolini SMMG, Junior JM. Morphophysiologial alterations resulted from the process of musculoskeletal system aging and its consequences for the human body. Perspectivas: biológicas e saúde. 2014;4:22-34
3. Järvinen TL, Sievänen H, Khan KM, Heinonen A, Kannus P. Shifting the focus in fracture prevention from osteoporosis to falls. BMJ. 2008;336(7636):124-6
4. World Health Organization. Global health and ageing. National Institute on Aging (NIA), National Institutes of Health (NIH). 2011:32 p. Available from: U.S. Department of Health and Human Services, Washington
5. World Health Organization [Internet]. Geneva: World Health Organization; c2015 [cited May 7, 2017]. Available from: http://www.who.int/.
6. Miljkovic N, Lim JY, Miljkovic I, Frontera WR. Aging of skeletal muscle fibers. Ann Rehabil Med. 2015;39(2):155-62
7. Beaudart C, Reginster JY, Slomian J, Buckinx F, Locquet M, Bruyère O. Prevalence of sarcopenia: the impact of different diagnostic cut-off limits. J Musculoskelet Neuronal Interact. 2014;14(4):425-31
8. Morley JE, Anker SD, Von haehling S. Prevalence, incidence, and clinical impact of sarcopenia: facts, numbers, and epidemiology-update 2014. J Cachexia Sarcopenia Muscle. 2014;5(4):253-9
9. Drey M, Krieger B, Sieber CC. et al. Motoneuron loss is associated with sarcopenia. J Am Med Dir Assoc. 2014;15(6):435-9
10. Sirola J, Kröger H. Similarities in acquired factors related to postmenopausal osteoporosis and sarcopenia. J Osteoporos. 2011;2011:536735
11. Cruz-jentoft AJ, Baeyens JP, Bauer JM. et al. Sarcopenia: European consensus on definition and diagnosis: Report of the European Working Group on Sarcopenia in Older People. Age Ageing. 2010;39(4):412-23
12. Rolland Y, Czerwinski S, Abellan van kan G. et al. Sarcopenia: its assessment, etiology, pathogenesis, consequences and future perspectives. J Nutr Health Aging. 2008;12(7):433-50
13. Morley JE. Sarcopenia: diagnosis and treatment. J Nutr Health Aging. 2008;12(7):452-6
14. Macintosh C, Morley JE, Chapman IM. The anorexia of aging. Nutrition. 2000;16(10):983-95
15. Neuwirth C, Burkhardt C, Alix J. et al. Quality Control of Motor Unit Number Index (MUNIX) Measurements in 6 Muscles in a Single-Subject "Round-Robin" Setup. PLoS ONE. 2016;11(5):e0153948
16. Kaya RD, Nakazawa M, Hoffman RL, Clark BC. Interrelationship between muscle strength, motor units, and aging. Exp Gerontol. 2013;48(9):920-5
17. Baumgartner RN, Waters DL, Gallagher D, Morley JE, Garry PJ. Predictors of skeletal muscle mass in elderly men and women. Mech Ageing Dev. 1999;107(2):123-36
18. Haren MT, Siddiqui AM, Armbrecht HJ. et al. Testosterone modulates gene expression pathways regulating nutrient accumulation, glucose metabolism and protein turnover in mouse skeletal muscle. Int J Androl. 2011;34(1):55-68
19. Alway SE, Myers MJ, Mohamed JS. Regulation of satellite cell function in sarcopenia. Front Aging Neurosci. 2014;6:246
20. Sinclair A, Morley JE, Rodriguez-mañas L. et al. Diabetes mellitus in older people: position statement on behalf of the International Association of Gerontology and Geriatrics (IAGG), the European Diabetes Working Party for Older People (EDWPOP), and the International Task Force of Experts in Diabetes. J Am Med Dir Assoc. 2012;13(6):497-502
21. Cesari M, Kritchevsky SB, Newman AB. et al. Added value of physical performance measures in predicting adverse health-related events: results from the Health, Aging And Body Composition Study. J Am Geriatr Soc. 2009;57(2):251-9
22. Newman AB, Kupelian V, Visser M. et al. Sarcopenia: alternative definitions and associations with lower extremity function. J Am Geriatr Soc. 2003;51(11):1602-9
23. Bassey EJ, Short AH. A new method for measuring power output in a single leg extension: feasibility, reliability and validity. Eur J Appl Physiol Occup Physiol. 1990;60(5):385-90
24. Lecker SH, Jagoe RT, Gilbert A. et al. Multiple types of skeletal muscle atrophy involve a common program of changes in gene expression. FASEB J. 2004;18(1):39-51
25. Muir SW, Montero-odasso M. Effect of vitamin D supplementation on muscle strength, gait and balance in older adults: a systematic review and meta-analysis. J Am Geriatr Soc. 2011;59(12):2291-300
26. Liu CK, Leng X, Hsu FC. et al. The impact of sarcopenia on a physical activity intervention: the Lifestyle Interventions and Independence for Elders Pilot Study (LIFE-P). J Nutr Health Aging. 2014;18(1):59-64
27. Smoliner C, Sieber CC, Wirth R. Prevalence of sarcopenia in geriatric hospitalized patients. J Am Med Dir Assoc. 2014;15(4):267-72
28. Rossi AP, Fantin F, Micciolo R. et al. Identifying sarcopenia in acute care setting patients. J Am Med Dir Assoc. 2014;15(4):303.e7-12
29. Masanes F, Culla A, Navarro-gonzalez M. et al. Prevalence of sarcopenia in healthy community-dwelling elderly in an urban area of Barcelona (Spain). J Nutr Health Aging. 2012;16(2):184-7
30. Wu IC, Lin CC, Hsiung CA. et al. Epidemiology of sarcopenia among community-dwelling older adults in Taiwan: a pooled analysis for a broader adoption of sarcopenia assessments. Geriatr Gerontol Int. 2014;14(Suppl 1):52-60
31. Kanis JA, Johnell O, Oden A, Johansson H, Mccloskey E. FRAX and the assessment of fracture probability in men and women from the UK. Osteoporos Int. 2008;19(4):385-97
32. Pearson OM, Lieberman DE. The aging of Wolff's "law": ontogeny and responses to mechanical loading in cortical bone. Am J Phys Anthropol. 2004(Suppl 39):63-99
33. Lauretani F, Bandinelli S, Bartali B. et al. Axonal degeneration affects muscle density in older men and women. Neurobiol Aging. 2006;27(8):1145-54
34. Qureshi AM, Mcguigan FE, Seymour DG, Hutchison JD, Reid DM, Ralston SH. Association between COLIA1 Sp1 alleles and femoral neck geometry. Calcif Tissue Int. 2001;69(2):67-72
35. Rivadeneira F, Houwing-duistermaat JJ, Beck TJ. et al. The influence of an insulin-like growth factor I gene promoter polymorphism on hip bone geometry and the risk of nonvertebral fracture in the elderly: the Rotterdam Study. J Bone Miner Res. 2004;19(8):1280-90
36. Van meurs JB, Rivadeneira F, Jhamai M. et al. Common genetic variation of the low-density lipoprotein receptor-related protein 5 and 6 genes determines fracture risk in elderly white men. J Bone Miner Res. 2006;21(1):141-50
37. Karasik D, Cohen-zinder M. The genetic pleiotropy of musculoskeletal aging. Front Physiol. 2012;3:303
38. Hein G, Weiss C, Lehmann G, Niwa T, Stein G, Franke S. Advanced glycation end product modification of bone proteins and bone remodelling: hypothesis and preliminary immunohistochemicalfindings. 2006. Ann. Rheum. Dis. 65, 101-104.
39. Khoury MJ, Mccabe LL, Mccabe ER. Population screening in the age of genomic medicine. N Engl J Med. 2003;348(1):50-8
40. Riggs BL, Khosla S, Melton LJ. Sex steroids and the construction and conservation of the adult skeleton. Endocr Rev. 2002;23(3):279-302
41. Watts NB, Adler RA, Bilezikian JP. et al. Osteoporosis in men: an Endocrine Society clinical practice guideline. J Clin Endocrinol Metab. 2012;97(6):1802-22
42. Cawthon PM, Harrison SL, Barrett-connor E. et al. Alcohol intake and its relationship with bone mineral density, falls, and fracture risk in older men. J Am Geriatr Soc. 2006;54(11):1649-57
43. Wright NC, Looker AC, Saag KG. et al. The recent prevalence of osteoporosis and low bone mass in the United States based on bone mineral density at the femoral neck or lumbar spine. J Bone Miner Res. 2014;29(11):2520-6
44. National Osteoporosis Foundation, “54 Million Americans Affected By Osteoporosis, Low Bone Mass” URL. https://www.nof.org/news/54-million-americans-affected-by-osteoporosis-and-low-bone-mass/. Accessed May 10. 2017
45. Bosy-westphal A, Müller MJ. Identification of skeletal muscle mass depletion across age and BMI groups in health and disease-there is need for a unified definition. Int J Obes (Lond). 2015;39(3):379-86
46. Janssen et al. 2002.
47. Studenski SA, Peters KW, Alley DE. et al. The FNIH sarcopenia project: rationale, study description, conference recommendations, and final estimates. J Gerontol A Biol Sci Med Sci. 2014;69(5):547-58
48. Mclean K, Day L, Dalton A. Economic evaluation of a group-based exercise program for falls prevention among the older community-dwelling population. BMC Geriatr. 2015;15:33. Kelly TL, Wilson KE, Heymsfield SB. Dual energy X-Ray absorptiometry body composition reference values from NHANES. PLoS ONE. 2009;4(9):e7038
49. Kelly TL, Wilson KE, Heymsfield SB. Dual energy X-Ray absorptiometry body composition reference values from NHANES. PLoS ONE. 2009;4(9):e7038
50. “2015 ISCD Official Positions - Adult. (n.d.)” URL: http://www.iscd.org/official-positions/2015-iscd-official-positions-adult/. Accessed May 12. 2017
51. Burgos Peláez R. [Global therapeutic approach to sarcopenia]. Nutr Hosp. 2006;21(Suppl 3):51-60
52. Wittert GA, Chapman IM, Haren MT, Mackintosh S, Coates P, Morley JE. Oral testosterone supplementation increases muscle and decreases fat mass in healthy elderly males with low-normal gonadal status. J Gerontol A Biol Sci Med Sci. 2003;58(7):618-25
53. Sakuma K, Yamaguchi A. Novel intriguing strategies attenuating to sarcopenia. J Aging Res. 2012;2012:251217
54. Thomas DR. Loss of skeletal muscle mass in aging: examining the relationship of starvation, sarcopenia and cachexia. Clin Nutr. 2007;26(4):389-99
55. Van geel TA, Geusens PP, Winkens B, Sels JP, Dinant GJ. Measures of bioavailable serum testosterone and estradiol and their relationships with muscle mass, muscle strength and bone mineral density in postmenopausal women: a cross-sectional study. Eur J Endocrinol. 2009;160(4):681-7
56. Brown M. Skeletal muscle and bone: effect of sex steroids and aging. Adv Physiol Educ. 2008;32(2):120-6
57. Roubenoff R. Catabolism of aging: is it an inflammatory process? Curr Opin Clin Nutr Metab Care. 2003;6(3):295-9
58. Sørensen MB, Rosenfalck AM, Højgaard L, Ottesen B. Obesity and sarcopenia after menopause are reversed by sex hormone replacement therapy. Obes Res. 2001;9(10):622-6
59. Brotto M, Abreu EL. Sarcopenia: pharmacology of today and tomorrow. J Pharmacol Exp Ther. 2012;343(3):540-6
60. Jørgensen JO, Vahl N, Hansen TB, Thuesen L, Hagen C, Christiansen JS. Growth hormone versus placebo treatment for one year in growth hormone deficient adults: increase in exercise capacity and normalization of body composition. Clin Endocrinol (Oxf). 1996;45(6):681-8
61. Svensson J, Sunnerhagen KS, Johannsson G. Five years of growth hormone replacement therapy in adults: age- and gender-related changes in isometric and isokinetic muscle strength. J Clin Endocrinol Metab. 2003;88(5):2061-9
62. Nieuwenhuizen WF, Weenen H, Rigby P, Hetherington MM. Older adults and patients in need of nutritional support: review of current treatment options and factors influencing nutritional intake. Clin Nutr. 2010;29(2):160-9
63. Ha L, Hauge T, Spenning AB, Iversen PO. Individual, nutritional support prevents undernutrition, increases muscle strength and improves QoL among elderly at nutritional risk hospitalized for acute stroke: a randomized, controlled trial. Clin Nutr. 2010;29(5):567-73
64. Bischoff-ferrari HA, Dawson-hughes B, Staehelin HB. et al. Fall prevention with supplemental and active forms of vitamin D: a meta-analysis of randomised controlled trials. BMJ. 2009;339:b3692
65. Calder PC. n-3 polyunsaturated fatty acids, inflammation, and inflammatory diseases. Am J Clin Nutr. 2006;83(6 Suppl):1505S-1519S
66. Kaiser M, Bandinelli S, Lunenfeld B. Frailty and the role of nutrition in older people. A review of the current literature. Acta Biomed. 2010;81(Suppl 1):37-45
67. Wolfe RR. Perspective: Optimal protein intake in the elderly. J Am Med Dir Assoc. 2013;14(1):65-6
68. Kim JS, Wilson JM, Lee SR. Dietary implications on mechanisms of sarcopenia: roles of protein, amino acids and antioxidants. J Nutr Biochem. 2010;21(1):1-13
69. Paddon-jones D, Rasmussen BB. Dietary protein recommendations and the prevention of sarcopenia. Curr Opin Clin Nutr Metab Care. 2009;12(1):86-90
70. Børsheim E, Bui QU, Tissier S, Kobayashi H, Ferrando AA, Wolfe RR. Effect of amino acid supplementation on muscle mass, strength and physical function in elderly. Clin Nutr. 2008;27(2):189-95
71. Johnston AP, De lisio M, Parise G. Resistance training, sarcopenia, and the mitochondrial theory of aging. Appl Physiol Nutr Metab. 2008;33(1):191-9
72. Strasser B, Keinrad M, Haber P, Schobersberger W. Efficacy of systematic endurance and resistance training on muscle strength and endurance performance in elderly adults-a randomized controlled trial. Wien Klin Wochenschr. 2009;121(23-24):757-64
73. Binder EF, Yarasheski KE, Steger-may K. et al. Effects of progressive resistance training on body composition in frail older adults: results of a randomized, controlled trial. J Gerontol A Biol Sci Med Sci. 2005;60(11):1425-31
74. Klitgaard H, Mantoni M, Schiaffino S. et al. Function, morphology and protein expression of ageing skeletal muscle: a cross-sectional study of elderly men with different training backgrounds. Acta Physiol Scand. 1990;140(1):41-54
75. National Osteoporosis Foundation, “Calcium, Vitamin D. What You Need to Know”. URL: https://www.nof.org/patients/treatment/calciumvitamin-d/get-the-facts-on-calcium-and-vitamin-d/. Accessed May 15. 2017
76. Judge J, Birge S, Gloth F 3rd, Heaney RP, Hollis BW, Kenny A, Kiel DP, Saliba D, Schneider DL, Vieth R. Recommendations abstracted from the American Geriatrics Society Consensus Statement on vitamin D for Prevention of Falls and Their Consequences. Journal of the American Geriatrics Society 62.1 (2013): 147-152.
77. University of Maryland Medical Center, “Osteoporosis”. URL: http://www.umm.edu/health/medical/reports/articles/osteoporosis. Accessed May 15. 2017
78. Cosman F, De beur SJ, Leboff MS. et al. Erratum to: Clinician's guide to prevention and treatment of osteoporosis. Osteoporos Int. 2015;26(7):2045-7
79. Granacher U, Gollhofer A, Hortobágyi T, Kressig RW, Muehlbauer T. The importance of trunk muscle strength for balance, functional performance, and fall prevention in seniors: a systematic review. Sports Med. 2013;43(7):627-41
80. Sherrington C, Whitney JC, Lord SR, Herbert RD, Cumming RG, Close JC. Effective exercise for the prevention of falls: a systematic review and meta-analysis. J Am Geriatr Soc. 2008;56(12):2234-43
81. Gillespie LD, Robertson MC, Gillespie WJ. et al. Interventions for preventing falls in older people living in the community. Cochrane Database Syst Rev. 2012(9):CD007146
82. Li F, Harmer P, Fisher KJ, McAuley E, Chaumeton N, Eckstrom E, Wilson NL. Tai Chi And Fall Reductions In Older Adults: A Randomized Controlled Trial. The Journals of Gerontology Series A: Biological Sciences and Medical Sciences 60.2 (2005): 187-194.
83. Cheung AM, Giangregorio L. Mechanical stimuli and bone health: what is the evidence? Curr Opin Rheumatol. 2012;24(5):561-66
84. Maurel DB, Boisseau N, Benhamou CL, Jaffre C. Alcohol and bone: Review of dose effects and mechanisms. Osteoporosis International. 2012;23(1):1-16
85. Heir T, Eide G. Injury proneness in infantry conscripts undergoing a physical training programme: smokeless tobacco use, higher age, and low levels of physical fitness are risk factors. Scand J Med Sci Sports. 1997;7(5):304-11
86. Kanis JA.World Health Organization Scientific Group. Assessment of osteoporosis at the primary health care level Technical Report Sheffield, United Kingdom: World Health Organization Collaborating Centre for Metabolic Bone Diseases. 2008.
87. Conti V, Russomanno G, Corbi G. et al. A polymorphism at the translation start site of the vitamin D receptor gene is associated with the response to anti-osteoporotic therapy in postmenopausal women from southern Italy. Int J Mol Sci. 2015;16(3):5452-66
88. Song J, Jin Z, Chang F, Li L, Su Y. Single and combined use of human parathyroid hormone (PTH) (1-34) on areal bone mineral density (aBMD) in postmenopausal women with osteoporosis: evidence based on 9 RCTs. Med Sci Monit. 2014;20:2624-32
89. Saag KG, Emkey R, Schnitzer TJ. et al. Alendronate for the prevention and treatment of glucocorticoid-induced osteoporosis. Glucocorticoid-Induced Osteoporosis Intervention Study Group. N Engl J Med. 1998;339(5):292-9
90. Black DM, Thompson DE, Bauer DC. et al. Fracture risk reduction with alendronate in women with osteoporosis: the Fracture Intervention Trial. FIT Research Group. J Clin Endocrinol Metab. 2000;85(11):4118-24
91. Boonen S, Reginster JY, Kaufman JM, Lippuner K, Zanchetta J, Langdahl B. et al. Fracture risk and zoledronic acid therapy in men with osteoporosis. N Engl J Med. 2012Nov;367(18):1714-23
92. Bethel M, Lohr KM, Carbone LD, Machua W, Diamond HS, Andary MT, Gellman H, Goldberg E, Hobar C, Jacobs-Kosmin D, Kaplan RJ, Lane JM, Lenrow D, Lin J, Moberg-Wolff E, Nalamachu SR, Salcido R, Schmitz MA, Serota AC, Shanmugam S, Slipman CW, Talavera F, Vuppalanchi S, Whyte II WS, Wiedel JD. Osteoporosis Treatment & Management. Medscape. Updated. 2016 Web
93. Rosen HN, Rosen CJ, Mulder JE. Selective estrogen receptor modulators for prevention and treatment of osteoporosis. UpToDate. 2015 Web
94. Riggs BL, Hartmann LC. Selective estrogen-receptor modulators - mechanisms of action and application to clinical practice. N Engl J Med. 2003;348:618
95. Pinkerton JV, Thomas S. Use of SERMs for treatment in postmenopausal women. J Steroid Biochem Mol Biol. 2014;142:142-54
96. Powles TJ, Hickish T, Kanis JA, Tidy A, Ashley S. Effect of tamoxifen on bone mineral density measured by dual-energy x-ray absorptiometry in healthy premenopausal and postmenopausal women. J Clin Oncol. 1996;14(1):78-84
97. Love RR, Mazess RB, Barden HS. et al. Effects of tamoxifen on bone mineral density in postmenopausal women with breast cancer. N Engl J Med. 1992;326(13):852-6
98. Ettinger B, Black DM, Mitlak BH. et al. Reduction of vertebral fracture risk in postmenopausal women with osteoporosis treated with raloxifene: results from a 3-year randomized clinical trial. Multiple Outcomes of Raloxifene Evaluation (MORE) Investigators. JAMA. 1999;282(7):637-45
99. Ronkin S, Northington R, Baracat E, Nunes MG, Archer DF, Constantine G. et al. Endometrial effects of bazedoxifene acetate, a novel selective estrogen receptor modulator, in postmenopausal women. ObstetGynecol. 2005;105(6):1397-404
100. Silverman SL, Christiansen C, Genant HK. et al. Efficacy of bazedoxifene in reducing new vertebral fracture risk in postmenopausal women with osteoporosis: results from a 3-year, randomized, placebo-, and active-controlled clinical trial. J Bone Miner Res. 2008;23(12):1923-34
101. Zhang X, Jeyakumar M, Petukhov S, Bagchi MK. A nuclear receptor corepressor modulates transcriptional activity of antagonist-occupied steroid hormone receptor. Mol Endocrinol. 1998;12(4):513-24
102. Couse JF, Korach KS. Estrogen receptor null mice: what have we learned and where will they lead us? Endocr Rev. 1999;20(3):358-417
103. Shang Y, Brown M. Molecular determinants for the tissue specificity of SERMs. Science. 2002;295(5564):2465-8
104. Tsai MJ, O'malley BW. Molecular mechanisms of action of steroid/thyroid receptor superfamily members. Annu Rev Biochem. 1994;63:451-86
105. Martinkovich S, Shah D, Planey SL, Arnott JA. Selective estrogen receptor modulators: tissue specificity and clinical utility. Clin Interv Aging. 2014;9:1437-52
106. Harada S, Rodan GA. Control of osteoblast function and regulation of bone mass. Nature. 2003;423(6937):349-55
107. Jilka RL. Molecular and cellular mechanisms of the anabolic effect of intermittent PTH. Bone. 2007;40(6):1434-46
108. Kneissel M, Boyde A, Gasser JA. Bone tissue and its mineralization in aged estrogen-depleted rats after long-term intermittent treatment with parathyroid hormone (PTH) analog SDZ PTS 893 or human PTH(1-34). Bone. 2001;28(3):237-50
109. Kraenzlin ME, Meier C. Parathyroid hormone analogues in the treatment of osteoporosis. Nat Rev Endocrinol. 2011;7(11):647-56
110. Chen P, Jerome CP, Burr DB. et al. Interrelationships between bone microarchitecture and strength in ovariectomized monkeys treated with teriparatide. J Bone Miner Res. 2007;22(6):841-8
111. Neer RM, Arnaud CD, Zanchetta JR. et al. Effect of parathyroid hormone (1-34) on fractures and bone mineral density in postmenopausal women with osteoporosis. N Engl J Med. 2001;344(19):1434-41
112. Kaufman JM, Orwoll E, Goemaere S. et al. Teriparatide effects on vertebral fractures and bone mineral density in men with osteoporosis: treatment and discontinuation of therapy. Osteoporos Int. 2005;16(5):510-6
113. Lindsay R, Scheele WH, Neer R. et al. Sustained vertebral fracture risk reduction after withdrawal of teriparatide in postmenopausal women with osteoporosis. Arch Intern Med. 2004;164(18):2024-30
114. Orwoll ES, Scheele WH, Paul S. et al. The effect of teriparatide [human parathyroid hormone (1-34)] therapy on bone density in men with osteoporosis. J Bone Miner Res. 2003;18(1):9-17
115. Martin TJ, Quinn JM, Gillespie MT, Ng KW, Karsdal MA, Sims NA. Mechanisms involved in skeletal anabolic therapies. Ann N Y Acad Sci. 2006;1068:458-70
116. Romito K, Herman CJ. Calcitonin for Osteoporosis. Osteoporosis Health Center. WebMD. 2014 Web
117. Aitken JM, Lindsay R, Hart DM. Long-term oestrogens for the prevention of post-menopausal osteoporosis. Postgrad Med J. 1976;52(Suppl 6):18-26
118. Christiansen C, Christensen MS, Transbøl I. Bone mass in postmenopausal women after withdrawal of oestrogen/gestagen replacement therapy. Lancet. 1981;1(8218):459-61
119. Wells G, Tugwell P, Shea B. et al. Meta-analyses of therapies for postmenopausal osteoporosis. V. Meta-analysis of the efficacy of hormone replacement therapy in treating and preventing osteoporosis in postmenopausal women. Endocr Rev. 2002;23(4):529-39
120. Yates J, Barrett-connor E, Barlas S, Chen YT, Miller PD, Siris ES. Rapid loss of hip fracture protection after estrogen cessation: evidence from the National Osteoporosis Risk Assessment. Obstet Gynecol. 2004;103(3):440-6
121. Cauley JA, Robbins J, Chen Z. et al. Effects of estrogen plus progestin on risk of fracture and bone mineral density: the Women's Health Initiative randomized trial. JAMA. 2003;290(13):1729-38
122. Rossouw JE, Anderson GL, Prentice RL. et al. Risks and benefits of estrogen plus progestin in healthy postmenopausal women: principal results From the Women's Health Initiative randomized controlled trial. JAMA. 2002;288(3):321-33
123. Moayyeri A. The association between physical activity and osteoporotic fractures: a review of the evidence and implications for future research. Ann Epidemiol. 2008;18(11):827-35
Author contact
Corresponding author: Carlos J. Padilla Colón, PhD; Mailing address: VA Caribbean Healthcare System (672), Research and Development Service (151), Room A-51b, # 10 Casia Street, San Juan, PR, 00921; Email: carlosjavier.padillacom; Telephone and fax numbers: 787-453-2354, 787-641-7582 extension 10177, FAX 787 641-4597
Received 2017-10-18
Accepted 2017-12-1
Published 2018-2-25