J Biomed 2018; 3:74-84. doi:10.7150/jbm.26783 This volume Cite
Review
Current Therapeutic Targets for Alzheimer's Disease
Department of Biotechnology, MNNIT, Allahabad, 211004
Abstract
Alzheimer's disease (AD) is one of the most common multifactorial diseases, including a range of abnormal cellular/molecular processes occurring in different regions of the brain. This disease is considered to be a major contributor to dementia in the elderly people. The pathophysiology involves accumulation of extracellular plaques containing the β-amyloid protein which is generated by the breakdown of the β-amyloid precursor protein (APP) in the brain. Another mechanism involves formation of intracellular neurofibrillary tangles of hyperphosphorylated tau protein. The AD can be classified into two types, familial AD (FAD) and sporadic AD (SAD) based on heritability apart from this the early-onset AD (EOAD) and late-onset AD (LOAD) forms are based on the age of onset. Some proteins, such as APOE, APP, BACE (b-amyloid cleaving enzyme), secretases, PS1/2 and tau proteins are reported in AD brain and have been correlated with disease. It is still unclear whether this disease comprises genetic or environmental factors or both. Many palliative drugs are available for the disease but there is still thirst for curative drugs with greater efficacy. It is required to understand the key factors involved in disease progression and their suitability as drug targets for discovering new drugs against Alzheimer's disease. Main purpose of this review is to highlight the potential targets for Alzheimer's disease that have been studied in recent years.
Keywords: Alzheimer's disease, Targets, Dementia, β-amyloid protein, Amyloid precursor protein, Genes.
Introduction
Today around 47 million people survive with dementia, globally. This number is projected to increase to more than 131 million by 2050 [1]. About 2.1 million Alzheimer's patients having age of 85 years or older were reported in year 2017[2]. Alzheimer's disease (AD) is one of the most common neurodegenerative disorders that causes dementia and it is characterized by amyloid deposition of a 39-42 AA peptide (Aβ) processed from the amyloid precursor protein (APP) and NFT. Genetic effects of APP and PSEN1/2 are also responsible for progression of the disease [3, 4, 5, 6] (Figure 1, 2). More than 95% cases of the AD are sporadic, where older age, low education degree, hypertension, hyperlipidaemia, heart disease; apolipoprotein E (ApoE) 4 allele polymorphism and diabetes are among the main factors responsible for the development of the disease. According to “amyloid cascade hypothesis” when processing of APP by β and γ-secretase forms Aβ40 and Aβ42 peptides which further undergo aggregation and oligomer formation and finally cause formation of Amyloid plaques. [7, 8].
Alzheimer's Drug Targets
Currently many targets are being considered for anti-Alzheimer's drug discovery. Some of these drug targets are already having known inhibitors while others are still being studied for designing suitable ligands against them. Such targets have been described in Table 1 along with their sources and known function.
β-Secretase: Aspartyl Proteases(BACE)
BACE is a novel target having 501 amino acids, a type 1 transmembrane aspartic protease, related to the pepsin and retroviral aspartic protease families. BACE is known to have highest expression level in human brain. BACE antisense oligonucleotide treatment to APP overexpressing cells is reportedly responsible for decreased production of β -secretase cleaved APP fragments. Recent studies suggest that levels of BACE1 protein and their activity was raised to approximately double in AD patient where BACE1 might initiate or enhance AD pathogenesis. The enzyme's key role in generation of Aβ, which is a major component of APP metabolism makes it popular target for drug development [9, 10, 11, 12, 13, 14].
Hypothetical model of AD pathophysiology (Pathophysiological pathway and Amyloidgenic pathway)
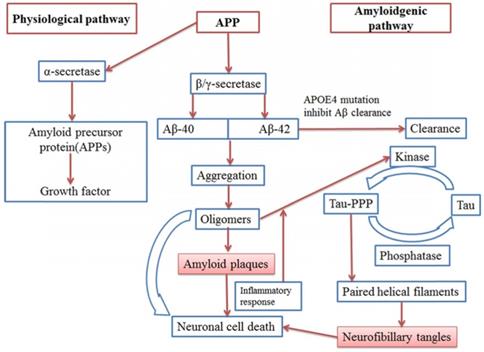
Pathophysiology and factors (Mutations, NFT, Oxidative stress, mitochondrial dysfunction) involved in AD
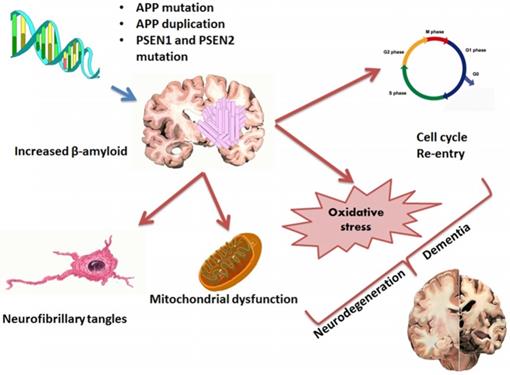
γ-Secretase: Presenilin I
γ-Secretase complex has 170kDa MW with an additional 30-70kDa MW derived from nicastrin glycosylation [15] reaching up to total size 230kDa with 19 TMs (Transmembrane-segment) that belong to the family of intramembrane cleaving protease, consist of Aspartyl protease, Zinc mettaloprotease site-2 protease family and serine protease. γ-Secretases are multi-subunit enzyme complexes having proteolytic activity and play a vital role in generation of Aβ [16,17].
Butyrylcholinesterase (BuChE)
Butyrylcholinesterase (BuChE) is a hydrolase which is responsible for hydrolysing esters of choline [18]. Degeneration of basal forebrain cholinergic system is an indication of the AD [19,20]. Studies have found that BuchE biochemical properties were changed in the neurodegenerative disease like in AD. Due to loss of neurons Ach and AchE levels expressed in the high amount which was responsible for the reduction of neurotransmitter and its enzyme [21, 22,23]. In cortical region BuchE level is increased during AD which is responsible for neuritic plaques and neurofibrillary tangle formation [19,24,25, 26,27,28,29].
Calcium-permeable AMPA receptors (CP-AMPARs)
AMPAR refers to one of the fastest excitatory neurotransmitters in the Central nervous system that are related to ionotropic glutamate receptors. It is involved in regulation of CP-AMARs in electrophysically produced synaptic plasticity and could be a therapeutic target for AD patients and other neurodegenerative disease. During Long-term potentiation (LTP) induction CP-AMPARs are employed from perisynaptic pools to contribute boosting synaptic Ca2+. [23].
Some researches suggests that CP-AMPAR is involved in the onset of synaptic pathology and formation of AMPAR and thus it is a therapeutic target for Alzheimer disease and other neurodegenerative Disease [30,31,32,33].
Calcitonin gene- regulated peptide (CGRP)
CGRP plays an important role as a potent vasodilator. It is known as a neurotransmitter in the Central nervous system [34] which contains 37 amino acids. It is distributed in different parts of the brain like hypothalamus, ventromedial nucleus of the thalamus, amygdala, grey matter, hippocampus and denate gyrate [35]. CGRP also helps in improving learning and memory processing [36].
Phosphodisterase (PDE)
PDE consist of a group of enzymes which control the rate of cAMP and cGMP hydrolysis and also contain 11 types of protein family members [37]. In brain regions like hippocampus, cortex stratium PDE isoforms play a crucial role in hydrolysis of cGMP [38,39,40,41,42,43,44] and intracellular signalling cascades. Studies suggest PDE2A, PDE5 as well as PDE9 are involved in memory formation [45, 43, 46, 47,48,49,50, 51, 52].
Muscarinic acetylcholine receptor (mAchR)
Muscarinic acetylcholine receptors (mAchR) belong to G-protein coupled muscarinic family and have some important functions like central cholinergic transmission learning and memory process [53,54]. M1 type of mAchR stimulates dephosphorylation of tau in PC12 cells which is responsible for alteration of hyperphosphorylation of tau protein and NFT pathology [55]. mAchR subtypes facilitate a variety of presynaptic and postsynaptic actions in hippocampus regions. In the hippocampus, presynaptic mAchRs reduces excitatory and inhibitory responses [56, 57, 58] and some studies suggest that different subtypes inhibit Aspartate, glutamate, g-Ambiobutyric acid and Acetylcholine [59]. Autoreceptors like M2, M2-cardiaclike and M2-non-cardiac like and M4 [60] [61] inhibit the Ach release in hippocampus [62, 63]. M1, M2 and M4 proteins are also found in forebrain region in case of AD patient [64]. M1 and M2 also play an important role in learning and memory process in other brain regions [65, 66, 67].
Dopamine 2 receptor
Dopamine 2 receptor belongs to GPCR family, involved in neural signaling that trigger many important behavioural processes. Dopamine which acts as major neurotransmitter is released by dopaminergic neurons to govern movement, cognition, and emotion in CNS. Studies performed on AD mouse model suggest that Levodopa, a chemical that is converted into dopamine in body, has protective effect in learning and memory process and also reduced Aβ plaques number and size [68, 69, 70].
Gama aminobutyric Acid A receptor (GABA)
In human nervous system (mainly CNS), GABA plays a crucial role as inhibitory neurotransmitter. GABA receptor directly act on membrane potentials via ionic, control short and long term neuronal activity, synaptic plasticity and network plasticity [71, 72, 73, 74, 75]. GABA and small proportion of somatostatin are used as a neurotransmitter in cerebral cortex [76]. Some evidence show that in post-mortem brain, GABA concentration declined in temporal, frontal and occipital lobes [77, 78, 79].
Nuclear factor E2 related factor-2 (Nrf2)
Nrf2 refers to a transcriptional activator of cell protection genes which also acts a therapeutic target for the treatment of neurodegenerative diseases including AD. Nrf2 targets contain cellular defence genes having drug metabolising enzymes, antioxidant response elements, DNA repair enzymes, molecular chaperons and proteasome subunits. These genes are involved in maintaining cellular redox balance and eliminating damaged proteins. Cellular stresses like oxidative damage was reportedly increased in case of AD [80,81]. Few studies suggest that xenobiotic metabolism is reduced in AD patients as well as APP/PS1 mutant mouse models [82,83]. Over expression of Nrf2 also protects against toxicity produced by Aβ 42 peptide in AD patients [84,85]. Nrf2 activity is regulated by Keap and GSK-3. Further GSK-3 plays a role in the pathogenesis of AD [86,87].
Gamma-secretase metabotropic glutamate receptor
Glutamate is known as primary excitatory neurotransmitter found in brain which activates G protein-coupled metabotropic glutamate receptors and ionotropic glutamate receptors [88]. Disruption in normal mGlu5 signalling is responsible for several neurodegenerative diseases like AD, Parkinson and Huntington disease [89,90]. mGluR5 binds to the heterotrimeric G-protein Gαq/11, which triggers phospholipase-C resulting in increased inositol-1,4,5 triphosphate formation and releasing of ca2+ from intracellular vesicles [91]. Studies suggest that mGluR5 also acts as receptor for Aβ42 and cellular prion protein (PrPc) [92,93,94,95,96,97].
N-myc downstream-regulated gene 2 (NDRG2)
N-myc downstream- regulated gene 2 is a family of genes having several functions like having role in differentiation, cell proliferation, and cell apoptosis. The NDRG comprises four members NDRG1-NDRG4. It has been observed that NDRG2 upregulation in AD models is involved in aging of brain [98, 99].
Serotonin 5-HT6 receptor
Serotonin 5HT6 was discovered in 1993 and studies suggest that 5-HT6 receptor has a crucial role in the targeting AD by improving cognition dysfunction, synaptic plasticity, neurogenesis and survival of neurons in adult brain. In AD patient 5HT6 receptor showed significant reduction in cortical areas. Blockage of 5-HT6 receptor induced acetylcholine release and also affects Gabaergic and Glutamergic systems. Further, it has also been found that dysregulation of the 5-HT6 receptor in temporal cortex may be associated with behavioural symptoms in AD [100,101].
Protein tyrosine phosphatase 1B (PTP1B)
PTP1B belongs to phosphatases involved in the relevant modulation of signalling pathways triggered by the initiation of the tyrosine kinase receptor family [102]. Studies suggested that PTP1B is involved in many important functions like learning, memory, endoplasmic reticulum, stress, regulation of synapse dynamics and microglial mediated neuroinflammation [103,104, 105,106;107]. Higher expression of PTP1B is observed in hippocampal microglial regions and it is involved positive regulation of neuroinflammation [108].
Monoamine oxidase B (MAO-B)
MAO is the type of enzyme found in an outer layer of mitochondrial membrane and plays a key role in the metabolism of monoamine neurotransmitter [109,110]. MAO-B has been reported in the pathogenesis of AD in the astrogila region. Upregulation of MAO-B is also responsible for the production of Reactive oxygen species (ROS) and H2O2 which leads to AD pathology [111]. MAO has two isoforms MAO-A and MAO-B [112]. Studies suggested that MAO-B expression is increased in different regions like the hippocampus, cerebral cortex and astrocyte contribute to the AD.
NAD(P)H Quinone oxidoreductase 1 (NQO1)
NAD(P)H Quinone oxidoreductase 1 is a type of redox regulated flavoenzyme which is also known as cytosolic homodimeric flavoprotein. It plays an important role in monitoring cellular redox state. NQO1 triggers two electron reductions of quinones and related molecule aimed to enhance their solubility and excretion. Reports suggest that NQO1 is involved in maintaining oxidative stress, abnormality in redox balance in AD patient [113; 114]. NQO1 could be a novel therapeutic target for the AD.
Neurotrophic Receptor Tyrosine Kinase 1 (NTRK1)
NTRK1 receptors are produced by NTRK1 gene which belong to the family of nerve growth factor receptors that contain neurotrophin as a ligand. Neurotrophin helps in regulation and development of CNS and PNS [115,116,117].Expression of NGF receptors of NGF receptors p75 (NTR) and TrkA occur in basal forebrain nucleus basalis (NB) which is responsible for the promotion of cell survival. These cells are degraded in the AD [118,119,120,53,121,122,123].
Amyloid protein precursor (APP)
Human APP gene was first discovered in 1987 from β-amyloid [124] and the gene was mapped to chromosome 21 [125,126]. APP belongs to membrane proteins containing extracellular domain and short cytoplasmic region. APP releases Aβ by two cleavage processes, one in the extracellular domain (β-secretase cleavage) and another one in the transmembrane region (γ-secretase cleavage). Different types of APP proteins can be formed by alternative splicing from the single gene. APP695 is a major splice form in neurons [127]. APP is cleaved by two different proteolytic pathways, one is a non-Amyloidogenic and other one is Amyloidogenic pathway. For this process, two main enzymes γ-secretase and β-secretase are responsible [128,129,130] (Figure 3).
Peroxisome proliferator activated receptor-γ(PPARs)
PPARs consist of nuclear hormone receptors that contain 48 human ligand inducible transcription factors. Their activity is regulated by lipid metabolites and steroids [131]. PPARs have three types of receptors PPAR-α, PPAR-β and PPAR-γ. PPARs are involved in many functions like lipid storage, adipocyte differentiation, lipid storage and glucose homeostasis in many organs like brain, adipose tissue, placenta and skin [132].Studies suggested that PPARs show a wide range of effect in AD pathophysiology through several animal models. PPAR- γ activation leads to an increase in APOE and ABCA1 levels in astrocytes and microglial cells [133,134,135,136,137,138,139,140,141,142,143].
Amyloidgenic process of APP hypothesis and formation of Aβ-42. APP protein can be processed by different enzymes like β-secretase, γ- secretase and α- secretase. sAPPβ and sAPPα is produced with peptides C83 and C99.
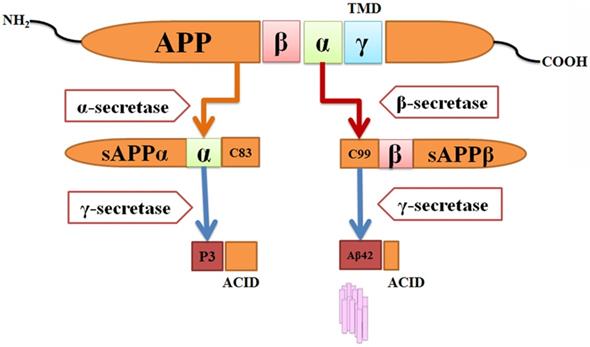
C-C chemokine receptor type-5
Chemokines belong to an expanding family of cytokines a(CXC), b(CC), g(C) and (CX3C) that are involved in the recruitment of leukocytes to inflammatory sites. Previous studies suggest that interleukin (IL-8) receptor B is found in cortical neurons, hippocampal and in neuritic plaques of AD brains [144,145,146,147,148,149,150].
Nicotinergic acetylcholine receptor (nAChR)
nAChRs, which belong to ligand-gated ion channels, comprise of five subunits. Higher expression of it's α-subunit is found in brain regions related to cognitive, memory function. nAChR is also involved in the progression of sensory information which shows the involvement of nAChr in the AD [151,152]. Some studies suggest that β-amyloid is responsible for triggering tau phosphorylation via several signalling pathways, free radical formation, lipid peroxidation, cell membrane damage and oxidative stress (153,154,155). It is also reported that α3 and α6 are also present in the brain [156,157]. Post-mortem brain sample studies showed the alteration of α4 & α7 nAchR in AD patients [158,159,160].
Angiotensin receptor
Angiotensin (AT) receptors, mainly AT1 receptor [161], play a vital role in peripheral activity and brain processes. Studies demonstrate that Angiotensin II receptor blockers (ARBs) are directly involved in neuroprotection in different part of brain like astrocyte, neurons, microglia and cerebrovascular endothelial cells [162]. ARBs are involved in many functions like anti-inflammatory compounds, inflammation triggered by glutamate excitotoxicity [163] and they also help in hippocampal synaptic signaling [164,165].
Non-amyloid-beta component precursor (NACP)
NACP is the type of presynaptic protein which is involved in amyloidogenesis and plaque formation [166,167,168,169,170]. NAC consists of long precursor (NACP) which produces NACP140 and NACP 112. [171,172]. Recent studies showed that NACP is a presynaptic nerve terminal protein [173,174,175] involved in neural plasticity, learning, and degeneration under the pathological condition in the AD.
c-Jun N-terminal kinases (JNK)
JNK which is also known as stress-activated protein kinase is triggered by exterior noxious stimuli via a kinase cascade [176; 177]. Activation of JNK phosphorylates serine-63 and 73 residues of C-jun and enhances transcription activity of AP-1 complex [178,179]. Studies suggested that JNK-3 has role in neuronal apoptosis, neural tube defects and oxidative stress in AD patient [180,181,182,183].
DJ-1/PARK7
DJ-1 is mostly expressed in mammals and forms a homodimer associated with neurodegenerative diseases. Some factors are involved in disruption of DJ-1 structure like monogenic mutants and chemical modification of cysteine residues. PARK7 gene is responsible for encoding of DJ-1 which has many functions like an antioxidant, molecular chaperon, protein degeneration and transcriptional regulation. DJ-1 has been reported for suppression of neurodegenerative diseases in the animal model. DJ-1 could be used as a therapeutic target for the AD [184].
Triggering receptor expressed on myeloid cells 2 (TREM2)
In the previous years, genetic studies have helped in the identification of variants in immune-related genes involved in the progression of AD [185]. Studies suggested that TREM2 have high-risk factors for developing of the AD. [186,187]. TREM2 is expressed on myeloid cells 2 (TREM2) which belong to immunoglobulin superfamily. Expression of TREM2 in the AD brain is primarily overexpressed on plaque-associated myeloid cells like Microglia, peripheral monocytes and brain-resident macrophages [188,189,190,191,192]. These cells are responsible for causing AD pathogenesis. Studies reported that TREM2 deficiency helps in reducing accumulation of myeloid cells around plaques and amyloid pathology in AD mouse model. [193,194,195].
Source and functions of all Alzheimer's disease therapeutic targets
S.No. | Name of target | Source | Functions | References |
---|---|---|---|---|
1. | β-Secretase | Astrocyte | Formation of Aβ | [9,10,11] |
2. | Butyrylcholinesterase | Basal forebrain | Neuritic plaques and neurofibrillary tangles | [19,20] |
3. | γ-Secretase: Presenilin I | Medial temporal lobe cortex | Formation of Aβ | [196] |
4. | CP-AMPARs | Hippocampi | Boost synaptic Ca2+ | [23] |
5. | Calcitonin gene- regulated peptide | Hypothalamus, ventromedial nucleus of the thalamus, amygdala, grey matter, hippocampus and denate gyrates | Neurotransmitter | [197] |
6. | Phosphodisterase (PDE) | Hippocampus, Cortex stratium | Hydrolysis of CGMP | [38,39,40,41,42,43,44] |
7. | Muscarinic acetylcholine receptor (mAchR) | Hippocampus | Hyperphosphorylation of tau protein | [56,58] |
8. | Dopamine 2 receptor | Central nervous system | Aβ plaques | [68,70] |
9. | Gama aminobutyric Acid A receptor | Cerebral cortex, temporal lobes, frontal and occipital lobes | Neurotransmitter in cerebral cortex | [71, 73,74,75,198] |
10. | Nuclear facto r E2 related factor-2 (Nrf2) | Temporal lobe, microglia and astrocytes | Antioxidant Maintaining redox balance and eliminate damage proteins | [80,81] |
11. | Gamma-secretase metabotropic glutamate receptor | Cortical and Hippocampal | Releasing of ca2+ | [91] |
12. | Parkinson disease protein 7(DJ-1/PARK7) | Hippocampus | Antioxidant, molecular chaperon, protein degeneration and transcriptional regulation | [184] |
13. | N-myc downstream-regulated gene 2 | Astrocytes, glia cells | Differentiation, cell proliferation and cell apoptosis | [199] |
14. | Serotonin 5-HT6 receptor | Cortical | Improving cognition dysfunction, synaptic plasticity | [100,101] |
15. | Protein tyrosine phosphatase 1B (PTP1B) | Hippocampal, microglial | Learning, memory, endoplasmic reticulum, stress, regulation of synapse dynamics and microglial mediated Neuroinflammation. | [108] |
16. | Monoamine oxidase B (MAO-B) | Astrogila, hippocampus, cerebral cortex and astrocyte | Metabolism of monoamine neurotransmitter | [111,112] |
17. | NAD (P)H Quinone oxidoreductase 1 | Oxidative stress, abnormality in redox balance | [113,114] | |
18. | Neurotrophic Receptor Tyrosine Kinase 1 | Basal forebrain Nucleus Basalis (NB) | Development of CNS and PNS, promotion of cell survival | [53,118,119,120, 121, 122,123] |
19. | Amyloid protein precursor (APP) | Hippocampus, olfactory bulb | Formation of Aβ | [200] |
20. | Peroxisome proliferator activated receptor-γ | Astrocytes and microglial cells | Lipid storage, adipocyte differentiation, and lipid storage and glucose homeostasis | [132] |
21. | C-C chemokine receptor type-5 | Cortical neurons, hippocampal | Recruitment of leukocytes to inflammatory sites | [144,145, 146,147,148, 149,150] |
22. | Nicotinergic acetylcholine receptor | Cerebral cortex | Cognitive, memory function and also involve in progression of sensory information | [151,152] |
23. | Angiotensin receptor | Astrocyte, neurons, microlia and cerebrovascular endothelial cells | Anti-inflammatory compounds synaptic signalling | [164,165] |
24. | Non-amyloid-beta component precursor | Neocortex, hippocampus, olfactory bulb, Striatum, thalamus, and cerebellum | Amyloidogenesis and plaque formation | [166,167,168,169,170] |
25. | c-Jun N-terminal kinases | Cortex hippocampus and cerebellum | Neuronal apoptosis, neural tube defects and oxidative stress | [201] |
26. | Triggering receptor expressed on myeloid cells 2 | Myeloid cells | amyloid pathology | [188,189,190] |
List of approved Alzheimer's drugs and their targets
S.No. | Approved drug | Target | References |
---|---|---|---|
27. | Donepezil | Acetylcholinesterase | [202,203, 204] |
28. | Rivastigmine | Acetylcholinesterase | |
29. | Galantamine | Acetylcholinesterase | |
30. | Memantine | NMDA receptor | [205] |
Conclusion
Dementia is increasing rapidly in world population. Alzheimer's disease is an important factor behind development of dementia in older people. Due to the unclearly known mechanism of pathophysiology and target identification Alzheimer's disease treatment remains as a great challenge for modern drug discovery. Only few targets and drugs are available for the treatment of the disease. In this review, we have focused on several known targets which are directly and indirectly involved in generation of amyloid beta and neurofibrillary tangle in AD as well as other molecules. These targets are found in different regions of the brain like Hippocampus, astrocyte, glial cells, temporal, frontal lobe, cortex, Striatum, thalamus, cerebellum and Basal forebrain Nucleus Basalis (NB). These parts of the brain have different types of functions like synaptic plasticity, Long-term potentiation, memory formation, oxidative stress, Neuronal apoptosis, Anti-inflammatory, cell survival etc. Finally the molecules that are involved in unbalancing normal functioning of these functions have been highlighted in this review. There is urgent need to explore these targets for designing efficient Alzheimer's drugs with minimum side effects.
Abbreviations
FAD: Familial Alzheimer's disease; SAD: Sporadic Alzheimer's disease; EOAD: Early Onset Alzheimer's disease; LOAD: Late-onset Alzheimer's disease; APOE: Apolipoprotein E; APP: Amyloid Precursor Protein; BACE: Beta-secretase; NFT: Neurofibrillary tangle; PSEN: Presenilin; BuchE: Butyrylcholinesterase; CP-AMPARs: Calcium-permeable AMPA receptors; AchE: Acetylcholinesterase; CGRP: Calcitonin gene-related peptide; CAMP: Cyclic adenosine monophosphate; CGMP: Cyclic guanosine monophosphate; PDE: Phosphodiesterase; mAchR: Muscarinic acetylcholine receptor; MAO-B: Monoamine oxidase B; NDRG2: N-myc downstream-regulated gene 2; PTP1B: Protein tyrosine phosphatase 1B; NADPHQ1: NAD (P)H Quinone oxidoreductase 1; NRTK1: Neurotrophic receptor tyrosine kinase 1; PPAR-γ: Peroxisome proliferator activated receptor-γ; nAchR: Nicotinergic acetylcholine receptor; TREM2: Triggering receptor expressed on myeloid cells 2; JNK: c-Jun N-terminal kinases; PARK7: Parkinson disease protein 7; ARBs: Angiotensin II receptor blockers; TMs: Transmembrane-segment.
Acknowledgements
AC and PKM are highly thankful to MNNIT Allahabad for PhD fellowship.
Author Contributions
AC and AM surveyed literature and prepared the manuscript. SS, BSY and PKM helped in writing the manuscript.
Competing Interests
The authors have declared that no competing interest exists.
References
1. Prince M, Comas-Herrera A, Knapp M, Guerchet M, Karagiannidou M. World Alzheimer report 2016: improving healthcare for people living with dementia: coverage, quality and costs now and in the future.
2. Hebert L. E, Weuve, J, Scherr, P. A, & Evans, D. A. Alzheimer disease in the United States (2010-2050) estimated using the 2010 census. Neurology. 2013:80 (19), 1778-1783
3. Selkoe DJ. Normal and abnormal biology of the beta-amyloid precursor protein. Annual review of neuroscience. 1994;17:489-517
4. Hardy J, Selkoe DJ. The amyloid hypothesis of Alzheimer's disease: progress and problems on the road to therapeutics. Science. 2002;297:353-6
5. Selkoe DJ, Hardy J. The amyloid hypothesis of Alzheimer9s disease at 25 years. EMBO molecular medicine. 2016;8:595-608
6. Selkoe DJ. Amyloid β protein precursor and the pathogenesis of Alzheimer's disease. Cell. 1989;58:611-2
7. Butterfield DA, Drake J, Pocernich C, Castegna A. Evidence of oxidative damage in Alzheimer's disease brain: central role for amyloid β-peptide. Trends in molecular medicine. 2001;7:548-54
8. Butterfield DA, Boyd-Kimball D. The critical role of methionine 35 in Alzheimer's amyloid β-peptide (1-42)-induced oxidative stress and neurotoxicity. Biochimica et Biophysica Acta (BBA)-Proteins and Proteomics. 2005;1703:149-56
9. Fukumoto H, Cheung BS, Hyman BT, Irizarry MC. β-Secretase protein and activity are increased in the neocortex in Alzheimer disease. Archives of neurology. 2002;59:1381-9
10. Yang LB, Lindholm K, Yan R, Citron M, Xia W, Yang XL, Beach T, Sue L, Wong P, Price D, Li R. Elevated β-secretase expression and enzymatic activity detected in sporadic Alzheimer disease. Nature medicine. 2003Jan1;9:3-4
11. Li Q, Südhof TC. Cleavage of amyloid-β precursor protein and amyloid-β precursor-like protein by BACE 1. Journal of Biological Chemistry. 2004;279:10542-50
12. Dingwall C. Spotlight on BACE: the secretases as targets for treatment in Alzheimer disease. Journal of Clinical Investigation. 2001;108:1243
13. Vassar R, Bennett BD, Babu-Khan S, Kahn S, Mendiaz EA, Denis P, Teplow DB, Ross S, Amarante P, Loeloff R, Luo Y. β-Secretase Cleavage of Alzheimer9s Amyloid Precursor Protein by the Transmembrane Aspartic Protease BACE. science. 1999Oct22;286:735-41
14. Sinha S, Anderson JP, Barbour R, Basi GS, Caccavello R, Davis D, Doan M, Dovey HF, Frigon N, Hong J, Jacobson-Croak K. Purification and cloning of amyloid precursor protein β-secretase from human brain. Nature. 1999;402:537-40
15. Schedin-Weiss S, Winblad B, Tjernberg LO. The role of protein glycosylation in Alzheimer disease. The FEBS journal. 2014;281:46-62
16. Brown MS, Ye J, Rawson RB, Goldstein JL. Regulated intramembrane proteolysis: a control mechanism conserved from bacteria to humans. Cell. 2000;100:391-8
17. Krishnaswamy S, Verdile G, Groth D, Kanyenda L, Martins RN. The structure and function of Alzheimer's gamma secretase enzyme complex. Critical reviews in clinical laboratory sciences. 2009;46:282-301
18. Silver A. Biology of cholinesterases. American Elsevier Pub. Co. 1974
19. Perry E, Perry R, Blessed G, Tomlinson B. Necropsy evidence of central cholinergic deficits in senile dementia. The Lancet. 1977;309:189
20. Geula C. & Mesulam, M. -M. in Alzheimer Disease (2nd edn) (eds Terry, R, Katzman, R, Bick, K. & Sisodia, S. S.) Lippincott Williams & Wilkins, Philadelphia, Pennsylvania. 1999:269-292
21. Geula C, Mesulam M. Special properties of cholinesterases in the cerebral cortex of Alzheimer's disease. Brain research. 1989;498:185-9
22. Arendt T, Brückner MK, Lange M, Bigl V. Changes in acetylcholinesterase and butyrylcholinesterase in Alzheimer's disease resemble embryonic development—a study of molecular forms. Neurochemistry international. 1992;21:381-96
23. Henley JM, Wilkinson KA. Synaptic AMPA receptor composition in development, plasticity and disease. Nature Reviews Neuroscience. 2016;17:337-50
24. Atack JR, Perry EK, Bonham JR, Candy JM, Perry RH. Molecular forms of acetylcholinesterase and butyrylcholinesterase in the aged human central nervous system. Journal of neurochemistry. 1986;47:263-77
25. Wright CI, Geula C, Mesulam M. Neuroglial cholinesterases in the normal brain and in Alzheimer's disease: relationship to plaques, tangles, and patterns of selective vulnerability. Annals of neurology. 1993Sep1;34:373-84
26. Moran M. A, Mufson, E. J, & GomezRamos, P. Colocalizatiori of cholinesterases with P-amyloid protein in aged and Alzheimer's brains. Acta Neuropathologica. 1993:85 362-369
27. Mesulam M, & Geula C. Butyrylcholinesterase reactivity differentiates the amyloid plaques of aging from those of dementia. Annals of Neurology. 1994:36 722-727
28. Geula C, Mesulam M. Cholinesterases and the pathology of Alzheimer disease. Alzheimer disease and associated disorders. 1995
29. Friede R. L. Enzyme histochemical studies of senile plaques. J. Neuropathol Exp. Neurol. 1965:24 477-91
30. Grooms SY, Opitz T, Bennett MV, Zukin RS. Status epilepticus decreases glutamate receptor 2 mRNA and protein expression in hippocampal pyramidal cells before neuronal death. Proceedings of the National Academy of Sciences. 2000;97:3631-6
31. Kwak S, Weiss JH. Calcium-permeable AMPA channels in neurodegenerative disease and ischemia. Current opinion in neurobiology. 2006;16:281-7
32. Rajasekaran K, Todorovic M, Kapur J. Calcium-permeable AMPA receptors are expressed in a rodent model of status epilepticus. Annals of neurology. 2012;72:91-102
33. Whitehead G, Jo J, Hogg EL, Piers T, Kim DH, Seaton G, Seok H, Bru-Mercier G, Son GH, Regan P, Hildebrandt L. Acute stress causes rapid synaptic insertion of Ca2+-permeable AMPA receptors to facilitate long-term potentiation in the hippocampus. Brain. 2013Dec1;136:3753-65
34. Van Rossum De, Hanisch Uk, Quirion R. Neuroanatomical localization, pharmacological characterization and functions of CGRP, related peptides and their receptors. Neuroscience & Biobehavioral Reviews. 1997Dec31;21:649-78
35. Skofitsch G, Jacobowitz DM. Autoradiographic distribution of 125 I calcitonin gene-related peptide binding sites in the rat central nervous system. Peptides. 1985;6:975-86
36. Kovács A, Telegdy G. Effects of intracerebroventricular administration of calcitonin gene-related peptide on passive avoidance behaviour in rats. Neuropeptides. 1992;23:51-4
37. Bender AT, Beavo JA. Cyclic nucleotide phosphodiesterases: molecular regulation to clinical use. Pharmacological reviews. 2006;58:488-20
38. Conti M, Jin SL. The molecular biology of cyclic nucleotide phosphodiesterases. Progress in nucleic acid research and molecular biology. 1999;63:1-38
39. Beavo JA, Brunton LL. Cyclic nucleotide research—still expanding after half a century. Nature reviews Molecular cell biology. 2002;3:710-8
40. Lakics V, Karran EH, Boess FG. Quantitative comparison of phosphodiesterase mRNA distribution in human brain and peripheral tissues. Neuropharmacology. 2010;59:367-74
41. Bolger GB, Rodgers L, Riggs M. Differential CNS expression of alternative mRNA isoforms of the mammalian genes encoding cAMP-specific phosphodiesterases Gene. 1994 Nov;149:237-44.
42. Repaske DR, Corbin JG, Conti M, Goy MF. A cyclic GMP-stimulated cyclic nucleotide phosphodiesterase gene is highly expressed in the limbic system of the rat brain. Neuroscience. 1993;56:673-86
43. Reyes-Irisarri E, Ittersum MV, Mengod G, De Vente J. Expression of the cGMP-specific phosphodiesterases 2 and 9 in normal and Alzheimer's disease human brains. European Journal of Neuroscience. 2007;25:3332-8
44. Van Staveren WC, Steinbusch HW, Ittersum MV, Repaske DR, Goy MF, Kotera J, Omori K, Beavo JA, De Vente J. mRNA expression patterns of the cGMP-hydrolyzing phosphodiesterases types 2, 5, and 9 during development of the rat brain. Journal of Comparative Neurology. 2003Dec22;467:566-80
45. Reneerkens OA, Rutten K, Steinbusch HW, Blokland A, Prickaerts J. Selective phosphodiesterase inhibitors: a promising target for cognition enhancement. Psychopharmacology. 2009;202:419-43
46. Van Der Staay FJ, Rutten K, Bärfacker L, DeVry J, Erb C, Heckroth H, Karthaus D, Tersteegen A, van Kampen M, Blokland A, Prickaerts J. The novel selective PDE9 inhibitor BAY 73-6691 improves learning and memory in rodents. Neuropharmacology. 2008Oct31;55:908-18
47. Boess FG, Hendrix M, van der Staay FJ, Erb C, Schreiber R, van Staveren W, de Vente J, Prickaerts J, Blokland A, Koenig G. Inhibition of phosphodiesterase 2 increases neuronal cGMP, synaptic plasticity and memory performance. Neuropharmacology. 2004;47:1081-92
48. Rodefer JS, Saland SK, Eckrich SJ. Selective phosphodiesterase inhibitors improve performance on the ED/ID cognitive task in rats. Neuropharmacology. 2012;62:1182-90
49. Van Der Staay, F. J, Rutten, K, Bärfacker, L, DeVry, J, Erb, C, Heckroth, H, .. & Prickaerts, J. The novel selective PDE9 inhibitor BAY 73-6691 improves learning and memory in rodents. Neuropharmacology. 2008 55(5), 908-918
50. Prickaerts J, Steinbusch HW, Smits JF, de Vente J. Possible role of nitric oxide-cyclic GMP pathway in object recognition memory: effects of 7-nitroindazole and zaprinast. European journal of pharmacology. 1997;337:125-36
51. Cuadrado-Tejedor M, Hervias I, Ricobaraza A, Puerta E, Perez-Roldan J. M, Garcia-Barroso, C, Franco, R, Aguirre, N, and Garcia-Osta, A. (2011) Sildenafil restores cognitive function without affecting Ass burden in an Alzheimer's disease mouse model. Br. J. Pharmacol 164. 2029 -41
52. García-Barroso C, Ricobaraza A, Pascual-Lucasa M, Unceta N, Rico A. J, Goicolea, M, Sallés, J, Lanciego, J, Oyarzabal, J, Franco, R, Cuadrado-Tejedor, M, and García-Osta, A. Tadalafil crosses the blood brain barrier and reverses cognitive dysfunction in a mouse model of AD. Neuropharmacology. 2012:64 114- 123
53. Coyle JT, Price DL, DeLong MR. Alzheimer's disease: a disorder of cortical cholinergic innervation. Science. 1983;219:1184-90
54. Bartus RT, Dean R3, Beer B, Lippa AS. The cholinergic hypothesis of geriatric memory dysfunction. Science. 1982;217:408-14
55. Sadot E, Gurwitz D, Barg J, Behar L, Ginzburg I, Fisher A. Activation of m1 muscarinic acetylcholine receptor regulates τ phosphorylation in transfected PC12 cells. Journal of neurochemistry. 1996;66:877-80
56. Valentino RJ, Dingledine R. Presynaptic inhibitory effect of acetylcholine in the hippocampus. Journal of Neuroscience. 1981;1:784-92
57. 57. Halliwell JV. Physiological mechanisms of cholinergic action in the hippocampus. Progress in brain research. 1990;84:255-72
58. Krnjević K. Central cholinergic mechanisms and function. Progress in brain research. 1993;98:285-2
59. Raiteri M, Marchi M, Paudice P. Presynaptic muscarinic receptors in the central nervous system. Annals of the New York Academy of Sciences. 1990;604:113-29
60. Richards MH. Rat hippocampal muscarinic autoreceptors are similar to the M2 (cardiac) subtype: comparison with hippocampal M1, atrial M2 and ileal M3 receptors. British journal of pharmacology. 1990;99:753-61
61. McKinney M, Miller J. H, & Aagaard, P. J. Pharmacological characterization of the rat hippocampal muscarinic autoreceptor. Journal of Pharmacology and Experimental Therapeutics. 1993;264:74-78
62. Lapchak PA, Araujo DM, Quirion R, Collier B. Binding sites for [3 H] AF-DX 116 and effect of AF-DX 116 on endogenous acetylcholine release from rat brain slices. Brain research. 1989;496:285-94
63. Mash DC, Flynn DD, Potter LT. Loss of M2 muscarine receptors in the cerebral cortex in Alzheimer's disease and experimental cholinergic denervation. Science. 1985;228:1115-8
64. Flynn DD, Ferrari-DiLeo G, Mash DC, Levey AI. Differential regulation of molecular subtypes of muscarinic receptors in Alzheimer's disease. Journal of neurochemistry. 1995;64:1888-91
65. Levey AI. Immunological localization of m1-m5 muscarinic acetylcholine receptors in peripheral tissues and brain. Life sciences. 1993;52:441-8
66. Levey AI, Edmunds SM, Koliatsos V, Wiley RG, Heilman CJ. Expression of m1-m4 muscarinic acetylcholine receptor proteins in rat hippocampus and regulation by cholinergic innervation. Journal of Neuroscience. 1995;15:4077-92
67. Rouse ST, Thomas TM, Levey AI. Muscarinic acetylcholine receptor subtype, m2: diverse functional implications of differential synaptic localization. Life sciences. 1997;60:1031-8
68. Blalock EM, Geddes JW, Chen KC, Porter NM, Markesbery WR, Landfield PW. Incipient Alzheimer's disease: microarray correlation analyses reveal major transcriptional and tumor suppressor responses. Proceedings of the National Academy of Sciences. 2004;101:2173-8
69. Ambrée O, Richter H, Sachser N, Lewejohann L, Dere E, de Souza Silva MA, Herring A, Keyvani K, Paulus W, Schäbitz WR. Levodopa ameliorates learning and memory deficits in a murine model of Alzheimer's disease. Neurobiology of aging. 2009;30:1192-204
70. Lidow MS, Goldman-Rakic PS, Rakic P, Innis RB. Dopamine D2 receptors in the cerebral cortex: distribution and pharmacological characterization with [3H] raclopride. Proceedings of the National Academy of Sciences. 1989;86:6412-6
71. Lüscher C, Malenka RC. NMDA receptor-dependent long-term potentiation and long-term depression (LTP/LTD). Cold Spring Harbor perspectives in biology. 2012;4:a005710
72. Nugent F. S, Niehaus, J. L, & Kauer, J. A. PKG and PKA signaling in LTP at GABAergic synapses. Neuropsychopharmacology. 2009:34 (7), 1829
73. Castillo PE, Chiu CQ, Carroll RC. Long-term plasticity at inhibitory synapses. Current opinion in neurobiology. 2011;21:328-38
74. Schultz LM. REVIEW: GABAergic Inhibitory Processes and Hippocampal Long-term Potentiation. The Neuroscientist. 1997;3:226-36
75. Inoue W, Baimoukhametova DV, Füzesi T, Cusulin JI, Koblinger K, Whelan PJ, Pittman QJ, Bains JS. Noradrenaline is a stress-associated metaplastic signal at GABA synapses. Nature neuroscience. 2013;16:605-12
76. Jones EG. Neurotransmitters in the cerebral cortex. Journal of neurosurgery. 1986;65:135-53
77. Rossor MN, Emson PC, Mountjoy CQ, Roth M, Iversen LL. Reduced amounts of immunoreactive somatostatin in the temporal cortex in senile dementia of Alzheimer type. Neuroscience letters. 1980;20:373-7
78. Ellison DW, Beal MF, Mazurek MF, Bird ED, Martin JB. A postmortem study of amino acid neurotransmitters in Alzheimer's disease. Annals of neurology. 1986;20:616-21
79. Sasaki H, Muramoto O, Kanazawa I, Arai H, Kosaka K, Iizuka R. Regional distribution of amino acid transmitters in postmortem brains of presenile and senile dementia of Alzheimer type. Annals of neurology. 1986;19:263-9
80. Nunomura A, Perry G, Aliev G, Hirai K, Takeda A, Balraj EK, Jones PK, Ghanbari H, Wataya T, Shimohama S, Chiba S. Oxidative damage is the earliest event in Alzheimer disease. Journal of Neuropathology & Experimental Neurology. 2001;60:759-67
81. Sultana R, Butterfield DA. Role of oxidative stress in the progression of Alzheimer's disease. Journal of Alzheimer's Disease. 2010;19:341-53
82. Ramsey C. P, Glass, C. A, Montgomery, M. B, Lindl, K. A, Ritson, G. P, Chia, L. A, .. & Jordan-Sciutto, K. L. Expression of Nrf2 in neurodegenerative diseases. Journal of Neuropathology & Experimental Neurology. 2007 66(1), 75-85
83. Sarlette A, Krampfl K, Grothe C, Neuhoff NV, Dengler R, Petri S. Nuclear erythroid 2-related factor 2-antioxidative response element signaling pathway in motor cortex and spinal cord in amyotrophic lateral sclerosis. Journal of Neuropathology & Experimental Neurology. 2008;67:1055-62
84. Kanninen K, Malm TM, Jyrkkänen HK, Goldsteins G, Keksa-Goldsteine V, Tanila H, Yamamoto M, Ylä-Herttuala S, Levonen AL, Koistinaho J. Nuclear factor erythroid 2-related factor 2 protects against beta amyloid. Molecular and Cellular Neuroscience. 2008;39:302-13
85. Llorens-Marítin M, Jurado J, Hernández F, Ávila J. GSK-3β, a pivotal kinase in Alzheimer disease. Frontiers in molecular neuroscience. 2014:7
86. Avrahami L, Farfara D, Shaham-Kol M, Vassar R, Frenkel D, Eldar-Finkelman H. Inhibition of Glycogen Synthase Kinase-3 Ameliorates β-Amyloid Pathology and Restores Lysosomal Acidification and Mammalian Target of Rapamycin Activity in the Alzheimer Disease Mouse Model IN VIVO AND IN VITRO STUDIES. Journal of Biological Chemistry. 2013;288:1295-306
87. Cuadrado A. Structural and functional characterization of Nrf2 degradation by glycogen synthase kinase 3/beta-TrCP. Free Radic Biol Med. 2015;88:147-57
88. Dhami GK, Ferguson SS. Regulation of metabotropic glutamate receptor signaling, desensitization and endocytosis. Pharmacology & therapeutics. 2006;111:260-71
89. M Ribeiro F, Paquet M, P Cregan S, SG Ferguson S. Group I metabotropic glutamate receptor signalling and its implication in neurological disease. CNS & Neurological Disorders-Drug Targets (Formerly Current Drug Targets-CNS & Neurological Disorders). 2010;9:574-95
90. Thathiah A, De Strooper B. The role of G protein-coupled receptors in the pathology of Alzheimer's disease. Nature Reviews Neuroscience. 2011;12:73-87
91. Ribeiro FM, DeVries RA, Hamilton A, Guimaraes IM, Cregan SP, Pires RG, Ferguson SS. Metabotropic glutamate receptor 5 knockout promotes motor and biochemical alterations in a mouse model of Huntington's disease. Human molecular genetics. 2013;23:2030-42
92. Um JW, Kaufman AC, Kostylev M, Heiss JK, Stagi M, Takahashi H, Kerrisk ME, Vortmeyer A, Wisniewski T, Koleske AJ, Gunther EC. Metabotropic glutamate receptor 5 is a coreceptor for Alzheimer aβ oligomer bound to cellular prion protein. Neuron. 2013;79:887-902
93. Beraldo FH, Arantes CP, Santos TG, Machado CF, Roffe M, Hajj GN, Lee KS, Magalhaes AC, Caetano FA, Mancini GL, Lopes MH. Metabotropic glutamate receptors transduce signals for neurite outgrowth after binding of the prion protein to laminin γ1 chain. The FASEB Journal. 2011;25:265-79
94. 94. Sokol DK, Maloney B, Long JM, Ray B, Lahiri DK. Autism, Alzheimer disease, and fragile X APP, FMRP, and mGluR5 are molecular links. Neurology. 2011;76:1344-52
95. Renner M, Lacor PN, Velasco PT, Xu J, Contractor A, Klein WL, Triller A. Deleterious effects of amyloid β oligomers acting as an extracellular scaffold for mGluR5. Neuron. 2010;66:739-54
96. Haas LT, Strittmatter SM. Oligomers of amyloid β prevent physiological activation of the cellular prion protein-metabotropic glutamate receptor 5 complex by glutamate in Alzheimer disease. Journal of Biological Chemistry. 2016;291:17112-21
97. Haas LT, Kostylev MA, Strittmatter SM. Therapeutic molecules and endogenous ligands regulate the interaction between brain cellular prion protein (PrPC) and metabotropic glutamate receptor 5 (mGluR5). Journal of Biological Chemistry. 2014;289:28460-77
98. Qu X, Zhai Y, Wei H, Zhang C, Xing G, Yu Y, & He F. Characterization and expression of three novel differentiation-related genes belong to the human NDRG gene family. Molecular and cellular biochemistry. 2002 229(1-2), 35-44
99. Zhou RH, Kokame K, Tsukamoto Y, Yutani C, Kato H, Miyata T. Characterization of the human NDRG gene family: a newly identified member, NDRG4, is specifically expressed in brain and heart. Genomics. 2001Apr1;73:86-97
100. Garcia-Alloza M, Hirst WD, Chen CL, Lasheras B, Francis PT, Ramirez MJ. Differential involvement of 5-HT1B/1D and 5-HT6 receptors in cognitive and non-cognitive symptoms in Alzheimer's disease. Neuropsychopharmacology. 2004;29:410
101. Marcos B, Chuang TT, Gil-Bea FJ, Ramirez MJ. Effects of 5-HT6 receptor antagonism and cholinesterase inhibition in models of cognitive impairment in the rat. British journal of pharmacology. 2008;155:434-40
102. Haj FG, Markova B, Klaman LD, Bohmer FD, Neel BG. Regulation of receptor tyrosine kinase signaling by protein tyrosine phosphatase-1B. Journal of Biological Chemistry. 2003;278:739-44
103. Vieira MN, Lyra e Silva NM, Ferreira ST, De Felice FG. Protein tyrosine phosphatase 1B (PTP1B): a potential target for Alzheimer's therapy? Frontiers in aging neuroscience. 2017;9:7
104. Zabolotny JM, Bence-Hanulec KK, Stricker-Krongrad A, Haj F, Wang Y, Minokoshi Y, Kim YB, Elmquist JK, Tartaglia LA, Kahn BB, Neel BG. PTP1B regulates leptin signal transduction in vivo. Developmental cell. 2002Apr30;2:489-95
105. Pandey NR, Zhou X, Qin Z, Zaman T, Gomez-Smith M, Keyhanian K, Anisman H, Brunel JM, Stewart AF, Chen HH. The LIM domain only 4 protein is a metabolic responsive inhibitor of protein tyrosine phosphatase 1B that controls hypothalamic leptin signaling. Journal of Neuroscience. 2013;33:12647-55
106. Bomfim T. R, Forny-Germano, L, Sathler, L. B, Brito-Moreira, J, Houzel, J. C, Decker, H, .. & Holscher, C. An anti-diabetes agent protects the mouse brain from defective insulin signaling caused by Alzheimer's disease-associated Aβ oligomers. The Journal of clinical investigation. 2012:122 (4), 1339-1353
107. Bonda DJ, Stone JG, Torres SL, Siedlak SL, Perry G, Kryscio R, Jicha G, Casadesus G, Smith MA, Zhu X, Lee HG. Dysregulation of leptin signaling in Alzheimer disease: evidence for neuronal leptin resistance. Journal of neurochemistry. 2014;128:162-72
108. Song GJ, Jung M, Kim JH, Park H, Rahman MH, Zhang S, Zhang ZY, Park DH, Kook H, Lee IK, Suk K. A novel role for protein tyrosine phosphatase 1B as a positive regulator of neuroinflammation. Journal of neuroinflammation. 2016;13:86
109. Cohen G, Kesler N. Monoamine oxidase and mitochondrial respiration. Journal of neurochemistry. 1999;73:2310-5
110. Cai Z. Monoamine oxidase inhibitors: promising therapeutic agents for Alzheimer's disease. Molecular medicine reports. 2014;9:1533-41
111. Borroni E, Bohrmann B, Grueninger F, Prinssen E, Nave S, Loetscher H, Chinta SJ, Rajagopalan S, Rane A, Siddiqui A, Ellenbroek B. Sembragiline: a novel, selective monoamine oxidase type B inhibitor for the treatment of Alzheimer's disease. Journal of Pharmacology and Experimental Therapeutics. 2017;362:413-23
112. Thomas T. Monoamine oxidase-B inhibitors in the treatment of Alzheimers disease. Neurobiology of aging. 2000;21:343-8
113. Chhetri J, King AE, Gueven N. Alzheimer's Disease and NQO1: Is there a Link? Current Alzheimer Research. 2018;15:56-66
114. Raina AK, Templeton DJ, Deak JC, Perry G, Smith MA. Quinone reductase (NQO1), a sensitive redox indicator, is increased in Alzheimer's disease. Redox Report. 1999;4:23-7
115. Bothwell M. p75NTR: a receptor after all. Science. 1996;272:506
116. Carter BD, Lewin GR. Neurotrophins live or let die: does p75 NTR decide? Neuron. 1997;18:187-90
117. Bibel M, Barde YA. Neurotrophins: key regulators of cell fate and cell shape in the vertebrate nervous system. Genes & development. 2000;14:2919-37
118. Counts SE, Nadeem M, Wuu J, Ginsberg SD, Saragovi HU, Mufson EJ. Reduction of cortical TrkA but not p75NTR protein in early-stage Alzheimer's disease. Annals of neurology. 2004;56:520-31
119. Mesulam M, Mufson EJ, Levey AI, Wainer BH. Cholinergic innervation of cortex by the basal forebrain: cytochemistry and cortical connections of the septal area, diagonal band nuclei, nucleus basalis (substantia innominata), and hypothalamus in the rhesus monkey. Journal of Comparative Neurology. 1983;214:170-97
120. Bartus RT. On neurodegenerative diseases, models, and treatment strategies: lessons learned and lessons forgotten a generation following the cholinergic hypothesis. Experimental neurology. 2000;163:495-29
121. DeKosky S. T, Harbaugh, R. E, Schmitt, F. A, Bakay, R. A. E, Chui, H. C, Knopman, D. S, .. & Markesbery, W. R. The Intraventricular Bethanecol Study Group: cortical biopsy in Alzheimer's disease—diagnostic accuracy and neurochemical, neuropathological, and cognitive correlations. Ann Neurol. 1992:32 625-632
122. Mufson EJ, Bothwell M, Kordower JH. Loss of nerve growth factor receptor-containing neurons in Alzheimer's disease: a quantitative analysis across subregions of the basal forebrain. Experimental neurology. 1989;105:221-32
123. Whitehouse PJ, Price DL, Clark AW, Coyle JT, DeLong MR. Alzheimer disease: evidence for selective loss of cholinergic neurons in the nucleus basalis. Annals of neurology. 1981Aug1;10:122-6
124. Kang J, Lemaire HG, Unterbeck A, Salbaum JM, Masters CL, Grzeschik KH, Multhaup G, Beyreuther K, Müller-Hill B. The precursor of Alzheimer's disease amyloid A4 protein resembles a cell-surface receptor. Nature. 1987;325:733-6
125. Goldgaber D, Lerman MI, McBride OW, Saffiotti U, Gajdusek DC. Characterization and chromosomal localization of a cDNA encoding brain amyloid of Alzheimer's disease. Science. 1987;235:877-81
126. Tanzi RE, Gusella JF, Watkins PC, Bruns GA, George-Hyslop PH, Van Keuren ML, Patterson D, Pagan S, Kurnit DM, Neve RL. Amyloid beta protein gene: cDNA, mRNA distribution, and genetic linkage near the Alzheimer locus. Science. 1987;235:880-5
127. Sandbrink R, Masters CL, Beyreuther K. APP gene family. Alternative splicing generates functionally related isoforms. Ann NYAcad Sci. 1996;777:281-7
128. Haass C. Take five—BACE and the γ-secretase quartet conduct Alzheimer9s amyloid β-peptide generation. The EMBO journal. 2004;23:483-8
129. Nilsberth C, Westlind-Danielsson A, Eckman CB, Condron MM, Axelman K, Forsell C, Stenh C, Luthman J, Teplow DB, Younkin SG, Näslund J. The'Arctic'APP mutation (E693G) causes Alzheimer's disease by enhanced Aβ protofibril formation. Nature neuroscience. 2001;4:887-93
130. Hendriks L, van Duijn CM, Cras P, Cruts M, Van Hul W, van Harskamp F, Warren A, McInnis MG, Antonarakis SE, Martin JJ, Hofman A. Presenile dementia and cerebral haemorrhage linked to a mutation at codon 692 of the β-amyloid precursor protein gene. Nature genetics. 1992;1:218-21
131. Kummer MP, Heneka MT. PPARs in Alzheimer's disease. PPAR research. 2008
132. Desvergne B, Wahli W. Peroxisome proliferator-activated receptors: nuclear control of metabolism. Endocrine reviews. 1999;20:649-88
133. De la Monte SM, Wands JR. Molecular indices of oxidative stress and mitochondrial dysfunction occur early and often progress with severity of Alzheimer's disease. Journal of Alzheimer's Disease. 2006;9:167-81
134. Heneka M. T, Landreth, G. E, & Feinstein, D. L. Role for peroxisome proliferator-activated receptor-γ in Alzheimer's disease. Annals of neurology. 2001;49:276-276
135. Landreth GE, Heneka MT. Anti-inflammatory actions of peroxisome proliferator-activated receptor gamma agonists in Alzheimer's disease. Neurobiology of aging. 2001;22:937-44
136. In'T Veld BA, Ruitenberg A, Hofman A, Launer LJ, van Duijn CM, Stijnen T, Breteler MM, Stricker BH. Nonsteroidal antiinflammatory drugs and the risk of Alzheimer's disease. New England Journal of Medicine. 2001;345:1515-21
137. Lehmann JM, Lenhard JM, Oliver BB, Ringold GM, Kliewer SA. Peroxisome proliferator-activated receptors α and γ are activated by indomethacin and other non-steroidal anti-inflammatory drugs. Journal of Biological Chemistry. 1997;272:3406-10
138. Yan Q, Zhang J, Liu H, Babu-Khan S, Vassar R, Biere AL, Citron M, Landreth G. Anti-inflammatory drug therapy alters β-amyloid processing and deposition in an animal model of Alzheimer's disease. Journal of Neuroscience. 2003Aug20;23:7504-9
139. Scacchi R, Pinto A, Gambina G, Rosano A, Corbo RM. The peroxisome proliferator-activated receptor gamma (PPAR-γ2) Pro12Ala polymorphism is associated with higher risk for Alzheimer's disease in octogenarians. Brain research. 2007;1139:1-5
140. Hamilton G, Proitsi P, Jehu L, Morgan A, Williams J, O'Donovan MC, Owen MJ, Powell JF, Lovestone S. Candidate gene association study of insulin signaling genes and Alzheimer's disease: evidence for SOS2, PCK1, and PPARγ as susceptibility loci. American Journal of Medical Genetics Part B: Neuropsychiatric Genetics. 2007;144:508-16
141. Koivisto A. M, Helisalmi, S, Pihlajamäki, J, Hiltunen, M, Koivisto, K, Moilanen, L, .. & Kesäniemi, Y. A. Association analysis of peroxisome proliferator-activated receptor gamma polymorphisms and late onset Alzheimer's disease in the Finnish population. Dementia and geriatric cognitive disorders. 2006;22:449-453
142. Sauder S, Kölsch H, Lütjohann D, Schulz A, Von Bergmann K, Maier W, Heun R. Influence of peroxisome proliferator-activated receptor γ gene polymorphism on 24S-hydroxycholesterol levels in Alzheimer's patients. Journal of neural transmission. 2005;112:1381-9
143. Forman BM, Chen J, Evans RM. Hypolipidemic drugs, polyunsaturated fatty acids, and eicosanoids are ligands for peroxisome proliferator-activated receptors alpha and delta. Proc Natl Acad Sci. 1997:94 (9), 4312-17
144. Premack BA, Schall TJ. Chemokine receptors: gateways to inflammation and infection. Nature medicine. 1996;2:1174-8
145. Baggiolini M, Dewald B, Moser B. Human chemokines: an update. Annual review of immunology. 1997;15:675-05
146. Bazan JF, Bacon KB, Hardiman G, Wang W, Soo K, Rossi D, Greaves DR, Zlotnik A, Schall TJ. A new class of membrane-bound chemokine with a CX3C motif. Nature. 1997;385:640-4
147. Xia M, Qin S, McNamara M, Mackay C, Hyman BT. Interleukin-8 receptor B immunoreactivity in brain and neuritic plaques of Alzheimer's disease. The American journal of pathology. 1997Apr;150:1267
148. Horuk R, Martin A. W, Wang, Z. X, Schweitzer, L, Gerassimides, A, Guo, H, & Parker, J. Expression of chemokine receptors by subsets of neurons in the central nervous system. The Journal of immunology. 1997;158:2882-2890
149. Horuk R, Martin A, Hesselgesser J, Hadley T, Lu ZH, Wang ZX, Peiper SC. The Duffy antigen receptor for chemokines: structural analysis and expression in the brain. Journal of leukocyte biology. 1996;59:29-38
150. Lavi E, Strizki JM, Ulrich AM, Zhang W, Fu L, Wang Q, O'Connor M, Hoxie JA, Gonzalez-Scarano F. CXCR-4 (Fusin), a co-receptor for the type 1 human immunodeficiency virus (HIV-1), is expressed in the human brain in a variety of cell types, including microglia and neurons. The American journal of pathology. 1997;151:1035
151. Vallés AS, Borroni MV, Barrantes FJ. Targeting brain α7 nicotinic acetylcholine receptors in Alzheimer's disease: rationale and current status. CNS drugs. 2014Nov1;28:975-87
152. D'andrea MR, Nagele RG, Wang HY, Peterson PA, Lee DH. Evidence that neurones accumulating amyloid can undergo lysis to form amyloid plaques in Alzheimer's disease. Histopathology;38:120-34.
153. Guan ZZ, Yu WF, Shan KR, Nordman T, Olsson J, Nordberg A. Loss of nicotinic receptors induced by beta-amyloid peptides in PC12 cells: Possible mechanism involving lipid peroxidation. Journal of neuroscience research. 2003;71:397-406
154. Pettit DL, Shao Z, Yakel JL. β-Amyloid1-42 peptide directly modulates nicotinic receptors in the rat hippocampal slice. Journal of Neuroscience. 2001Jan1;21:RC120 -
155. Guan ZZ, Zhang X, Ravid R, Nordberg A. Decreased protein levels of nicotinic receptor subunits in the hippocampus and temporal cortex of patients with Alzheimer's disease. Journal of neurochemistry. 2000;74:237-43
156. O Neill MJ, Murray TK, Lakics V, Visanji NP, Duty S. The role of neuronal nicotinic acetylcholine receptors in acute and chronic neurodegeneration. CURRENT DRUG TARGETS-CNS AND NEUROLOGICAL DISORDERS. 2002;1:399-412
157. Perez XA, Bordia T, McIntosh JM, Quik M. α6β2* and α4β2* nicotinic receptors both regulate dopamine signaling with increased nigrostriatal damage: relevance to Parkinson's disease. Molecular pharmacology. 2010;78:971-80
158. Perry EK, Morris CM, Court JA, Cheng A, Fairbairn AF, McKeith IG, Irving D, Brown A, Perry RH. Alteration in nicotine binding sites in Parkinson's disease, Lewy body dementia and Alzheimer's disease: possible index of early neuropathology. Neuroscience. 1995;64:385-95
159. Hellström-Lindahl E, Mousavi M, Zhang X, Ravid R, Nordberg A. Regional distribution of nicotinic receptor subunit mRNAs in human brain: comparison between Alzheimer and normal brain. Molecular brain research. 1999;66:94-103
160. Burghaus L, Schütz U, Krempel U, de Vos RA, Steur EN, Wevers A, Lindstrom J, Schröder H. Quantitative assessment of nicotinic acetylcholine receptor proteins in the cerebral cortex of Alzheimer patients. Molecular Brain Research. 2000;76:385-8
161. Wu X, Kihara T, Hongo H, Akaike A, Niidome T, Sugimoto H. Angiotensin receptor type 1 antagonists protect against neuronal injury induced by oxygen-glucose depletion. British journal of pharmacology. 2010Sep1;161:33-50
162. Saavedra JM. Evidence to consider angiotensin II receptor blockers for the treatment of early Alzheimer's disease. Cellular and molecular neurobiology. 2016;36:259-79
163. Wang J, Pang T, Hafko R, Benicky J, Sanchez-Lemus E, Saavedra JM. Telmisartan ameliorates glutamate-induced neurotoxicity: roles of AT 1 receptor blockade and PPARγ activation. Neuropharmacology. 2014;79:249-61
164. Sohn YI, Lee NJ, Chung A, Saavedra JM, Turner RS, Pak DT, Hoe HS. Antihypertensive drug Valsartan promotes dendritic spine density by altering AMPA receptor trafficking. Biochemical and biophysical research communications. 2013;439:464-70
165. Kurinami H, Shimamura M, Sato N, Nakagami H, & Morishita R. Do angiotensin receptor blockers protect against Alzheimer's disease? Drugs & aging. 2013;30:367-372
166. Masliah E, Iwai A, Mallory M, Uéda K, Saitoh T. Altered presynaptic protein NACP is associated with plaque formation and neurodegeneration in Alzheimer's disease. The American journal of pathology. 1996;148:201
167. Irizarry MC, Kim TW, McNamara M, Tanzi RE, George JM, Clayton DF, Hyman BT. Characterization of the precursor protein of the non-Aβ component of senile plaques (NACP) in the human central nervous system. Journal of Neuropathology & Experimental Neurology. 1996;55:889-95
168. Iwai A, Masliah E, Yoshimoto M, Ge N, Flanagan L, De Silva HR, Kittel A, Saitoh T. The precursor protein of non-Aβ component of Alzheimer's disease amyloid is a presynaptic protein of the central nervous system. Neuron. 1995;14:467-75
169. Han H, Weinreb PH, Lansbury PT. The core Alzheimer's peptide NAC forms amyloid fibrils which seed and are seeded by β-amyloid: is NAC a common trigger or target in neurodegenerative disease? Chemistry & biology. 1995;2:163-9
170. Yoshimoto M, Iwai A, Kang D, Otero DA, Xia Y, Saitoh T. NACP, the precursor protein of the non-amyloid beta/A4 protein (A beta) component of Alzheimer disease amyloid, binds A beta and stimulates A beta aggregation. Proceedings of the National Academy of Sciences. 1995Sep26;92:9141-5
171. Uéda K, Fukushima H, Masliah E, Xia Y, Iwai A, Yoshimoto M, Otero DA, Kondo J, Ihara Y, Saitoh T. Molecular cloning of cDNA encoding an unrecognized component of amyloid in Alzheimer disease. Proceedings of the National Academy of Sciences. 1993;90:11282-6
172. Ueda K, Saitoh T, Mori H. Tissue-dependent alternative splicing of mRNA for NACP, the precursor of non-Aβ component of Alzheimer′ s disease amyloid. Biochemical and biophysical research communications. 1994;205:1366-72
173. Jakes R, Spillantini MG, Goedert M. Identification of two distinct synucleins from human brain. FEBS letters. 1994;345:27-32
174. George JM, Jin H, Woods WS, Clayton DF. Characterization of a novel protein regulated during the critical period for song learning in the zebra finch. Neuron. 1995;15:361-72
175. Iwai A, Yoshimoto M, Masliah E, & Saitoh T. Non-A. beta. Component of Alzheimer's Disease Amyloid (NAC) is Amyloidogenic. Biochemistry. 1995;34:10139-10145
176. Davis R. J. Signal transduction by the JNK group of MAP kinases. In Inflammatory Processes. 2000:13-21
177. Chang L, Karin M. Mammalian MAP kinase signalling cascades. Nature. 2001;410:37-40
178. Dérijard B, Hibi M, Wu IH, Barrett T, Su B, Deng T, Karin M, Davis RJ. JNK1: a protein kinase stimulated by UV light and Ha-Ras that binds and phosphorylates the c-Jun activation domain. Cell. 1994;76:1025-37
179. Kyriakis JM, Banerjee P, Nikolakaki E, Dai T, Rubie EA, Ahmad MF, Avruch J, Woodgett JR. The stress-activated protein kinase subfamily of c-Jun kinases. Nature. 1994;369:156-60
180. Kuan CY, Yang DD, Roy DR, Davis RJ, Rakic P, Flavell RA. The Jnk1 and Jnk2 protein kinases are required for regional specific apoptosis during early brain development. Neuron. 1999;22(4):667-6
181. Yang DD, Kuan CY, Whitmarsh AJ, Rinócn M, Zheng TS, Davis RJ, Rakic P, Flavell RA. Absence of excitotoxicity-induced apoptosis in the hippocampus of mice lacking the Jnk3 gene. Nature. 1997Oct23;389:865-70
182. Sabapathy K, Jochum W, Hochedlinger K, Chang L, Karin M, Wagner EF. Defective neural tube morphogenesis and altered apoptosis in the absence of both JNK1 and JNK2. Mechanisms of development. 1999;89:115-24
183. Yarza R, Vela S, Solas M, Ramirez MJ. c-Jun N-terminal kinase (JNK) signaling as a therapeutic target for Alzheimer's disease. Frontiers in pharmacology. 2016Jan12;6:321
184. Hijioka M, Inden M, Yanagisawa D, & Kitamura Y. DJ-1/PARK7: A New Therapeutic Target for Neurodegenerative Disorders. Biological and Pharmaceutical Bulletin. 2017;40:548-552
185. Guerreiro R, Wojtas A, Bras J, Carrasquillo M, Rogaeva E, Majounie E, Cruchaga C, Sassi C, Kauwe JS, Younkin S, Hazrati L. TREM2 variants in Alzheimer's disease. New England Journal of Medicine. 2013;368:117-27
186. Jonsson T, Stefansson H, Steinberg S, Jonsdottir I, Jonsson PV, Snaedal J, Bjornsson S, Huttenlocher J, Levey AI, Lah JJ, Rujescu D. Variant of TREM2 associated with the risk of Alzheimer's disease. New England Journal of Medicine. 2013;368:107-16
187. Lill CM, Rengmark A, Pihlstrøm L, Fogh I, Shatunov A, Sleiman PM, Wang LS, Liu T, Lassen CF, Meissner E, Alexopoulos P. The role of TREM2 R47H as a risk factor for Alzheimer's disease, frontotemporal lobar degeneration, amyotrophic lateral sclerosis, and Parkinson's disease. Alzheimer's & Dementia. 2015;11:1407-16
188. Jay TR, Miller CM, Cheng PJ, Graham LC, Bemiller S, Broihier ML, Xu G, Margevicius D, Karlo JC, Sousa GL, Cotleur AC. TREM2 deficiency eliminates TREM2+ inflammatory macrophages and ameliorates pathology in Alzheimer's disease mouse models. Journal of Experimental Medicine. 2015 jem-20142322
189. Frank M. C, Everett, D. L, Fedorenko, E, & Gibson, E. Number as a cognitive technology: Evidence from Pirahã language and cognition. Cognition. 2008;108:819-4
190. Melchior B, Garcia AE, Hsiung BK, Lo KM, Doose JM, Thrash JC, Stalder AK, Staufenbiel M, Neumann H, Carson MJ. Dual induction of TREM2 and tolerance-related transcript, Tmem176b, in amyloid transgenic mice: implications for vaccine-based therapies for Alzheimer's disease. ASN neuro. 2010;2:AN20100010
191. El Khoury J, Toft M, Hickman SE, Means TK, Terada K, Geula C, Luster AD. Ccr2 deficiency impairs microglial accumulation and accelerates progression of Alzheimer-like disease. Nature medicine. 2007;13:432-8
192. Gate D, Rezai-Zadeh K, Jodry D, Rentsendorj A, Town T. Macrophages in Alzheimer's disease: the blood-borne identity. Journal of neural transmission. 2010;117:961-70
193. Koronyo Y, Salumbides BC, Sheyn J, Pelissier L, Li S, Ljubimov V, Moyseyev M, Daley D, Fuchs DT, Pham M, Black KL. Therapeutic effects of glatiramer acetate and grafted CD115+ monocytes in a mouse model of Alzheimer's disease. Brain. 2015;138:2399-422
194. Ulrich JD, Finn MB, Wang Y, Shen A, Mahan TE, Jiang H, Stewart FR, Piccio L, Colonna M, Holtzman DM. Altered microglial response to Aβ plaques in APPPS1-21 mice heterozygous for TREM2. Molecular neurodegeneration. 2014;9:20
195. Tanzi RE. TREM2 and risk of Alzheimer's disease—Friend or foe? New England Journal of Medicine. 2015;372:2564-5
196. Pluta R, Kocki J, Ułamek-Kozioł M, Bogucka-Kocka A, Gil-Kulik P, Januszewski S, Jabłoński M, Petniak A, Brzozowska J, Bogucki J, Furmaga-Jabłońska W. Alzheimer-associated presenilin 2 gene is dysregulated in rat medial temporal lobe cortex after complete brain ischemia due to cardiac arrest. Pharmacological Reports. 2016;68:155-61
197. Park P, Sanderson TM, Amici M, Choi SL, Bortolotto ZA, Zhuo M, Kaang BK, Collingridge GL. Calcium-permeable AMPA receptors mediate the induction of the protein kinase A-dependent component of long-term potentiation in the hippocampus. Journal of Neuroscience. 2016;36:622-31
198. Nugent FS, Penick EC, Kauer JA. Opioids block long-term potentiation of inhibitory synapses. Nature. 2007;446:1086-90
199. Flügge G, Araya-Callis C, Garea-Rodriguez E, Stadelmann-Nessler C, Fuchs E. NDRG2 as a marker protein for brain astrocytes. Cell and tissue research. 2014Jul1;357(1):31-41
200. Maldonado TA, Jones RE, Norris DO. Distribution of β-amyloid and amyloid precursor protein in the brain of spawning (senescent) salmon: a natural, brain-aging model. Brain research. 2000;858:237-51
201. Coffey ET. Nuclear and cytosolic JNK signalling in neurons. Nature Reviews Neuroscience. 2014;15:285-99
202. Venneri A, Lane R. Effects of cholinesterase inhibition on brain white matter volume in Alzheimer's disease. Neuroreport. 2009Feb18;20:285-8
203. Muthuraju S, Maiti P, Solanki P, Sharma AK, Singh SB, Prasad D, Ilavazhagan G. Acetylcholinesterase inhibitors enhance cognitive functions in rats following hypobaric hypoxia. Behavioural brain research. 2009;203:1-4
204. Giacobini E. Cholinesterases: new roles in brain function and in Alzheimer's disease. Neurochemical research. 2003;28:515-22
205. Danysz W, Parsons CG. The NMDA receptor antagonist memantine as a symptomatological and neuroprotective treatment for Alzheimer's disease: preclinical evidence. International journal of geriatric psychiatry. 2003:18 (S1)
Author contact
Corresponding author: Dr. Ashutosh Mani, Department of Biotechnology, MNNIT, Allahabad, 211004. E-mail: amanicom
Received 2018-4-20
Accepted 2018-6-9
Published 2018-9-5