J Biomed 2019; 4:1-6. doi:10.7150/jbm.30269 This volume Cite
Research Paper
Disrupted fluid balance and baroreflex sensitivity in acute aortic regurgitation
1. Department of Physiology, Institute of Bioscience, São Paulo State University, UNESP-Botucatu, SP, Brazil
2. Department of Medicine, Federal University of São Carlos, SP, Brazil
Received 2018-9-28; Accepted 2018-11-12; Published 2019-1-23
Abstract
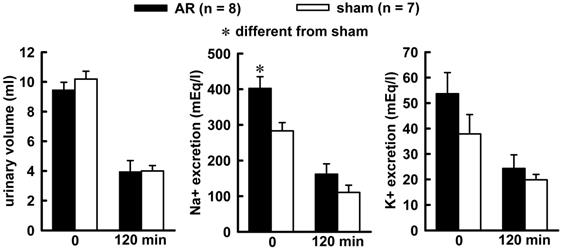
Acute aortic regurgitation (AR) causes abrupt volume overload to the heart. The implication of this acute volume overload concerning fluid balance and autonomic participation remains unknown. We studied fluid balance (sodium and water intake and excretion), autonomic modulation and heart rate variability (HRV) in acute AR rats. Male Wistar rats (260-280g) were submitted to sham or AR surgery by retrograde puncture of the aortic valves leaflets. The presence and severity of AR was confirmed by echocardiography exams one week after the surgeries. The left ventricule diastolic diameter and the left atrium area were bigger in acute AR than in sham rats. The fluid behavior was challenged by combining furosemide and captopril in low doses. This combined treatment induces water and sodium intake behavior within one hour. There was an increase in water intake and natriureses following a fluid depletion in acute AR rats. The daily intake and natriuresis were not altered. The diastolic arterial pressure was lower in AR than in sham and there were no changes in autonomic modulation. The study of HRV shows an increase in the high-frequency component in acute AR rats. However, there was a decrease in the spontaneous baroreflex sensitivity in these rats. In conclusion, the results show that an acute volume overload to the heart impairs since its onset not only the fluid balance but also baroreflex sensitivity.
Keywords: acute aortic regurgitation, baroreflex sensitivity, natriureses, volume overload, water intake
Introduction
Aortic regurgitation (AR) occurs due to the malfunction of the leaflets of the aortic valve producing large flow of blood back to the left ventricule (LV)1,2,3. Acute AR determines poor prognostic, usually a surgical emergency. The abrupt volume overload to the heart increases LV pressure in a non-prepared heart, causing acute pulmonary congestion3,4,5. After the critical period of overload, patients can remain asymptomatic for long periods, even with the hypertrophied heart1,2,3,6. Experimentally, in two weeks of AR (an acute phase) the heart already presents an augment of gene expression of a variety of extracellular matrix components thus induces fibroses in the LV, and eventually hypertrophy of the heart7,8,9.
The fluid and excretion balance in this situation has not been evaluated yet. It is well known that the heart contains several receptors sensitive to changes in blood volume10,11,12,13 which influence fluid balance and autonomic modulation to circulatory system. In healthy animals receptors close to the heart monitors increases in circulating volume and, consequently, inhibits fluid intake12,13. Indeed, those receptors might be also involved in activating reflexes to the circulation leading to diuresis in order to deal with this extra volume10,14,15. Previous experiments addressed to volume overload into the cardiovascular system infused volume into the circulation or simulated blood expansion, which affect other receptors all over the body, e.g. receptors in the kidneys and liver10,14. The AR model of overload to the heart allows to study mainly the role of cardiac receptors during volume expansion. It is well known that in late stages of volume overload to the heart, such as heart failure, the fluid balance is disrupted, and patients may develop swelling6. On the other hand, in the very early stages of developing volume overload to the heart there is nothing regarding the fluid balance.
The study of the heart rate variability (HRV) helps to identify heart rate fluctuations in different situations, and it gives an idea about the modulation of sympathetic and parasympathetic activity to the heart16,17. The HRV is divided into the high frequency (HF) component, believed to be from parasympathetic drive, and into the low frequency (LF) component, which might reflect the sympathetic drive to the heart16,17. Moreover, the study of the baroreflex sensitivity (BRS) helps also to access the autonomic modulation to the heart18,19. It is worth investigating how these variables would be after an acute exposure of the heart to a volume overload. This information might unveil the onset effects of volume overload to the heart. Therefore, in the present study we explore how the fluid intake and excretion, as well as the HRV and BRS would be in a model of acute AR in rats.
Results
Echocardiographic data
Echocardiograms variables for acute AR rats are showed in Table 1. The parameters revealed that the heart was exposed to an overload and was hypertrophied. Left ventricle diastolic diameter, the normalized left atrium diameter and area were bigger in AR than in sham rats, Table 1.
Daily measures
Daily fluid intake was measured over one week and presented no differences between the groups (water intake: 36.0 ± 2.7 vs sham 32.4 ± 3.6 ml/24 h, p = 0.364; 0.3 M NaCl intake: 4.3 ± 1.3 vs sham 5.6 ± 1.6 ml/24 h, p = 0.475).
Acute fluid depletion
Combined treatment of Furosemide (Furo) plus captopril (Cap) produced an increase in water (8.7 ± 0.6vs sham 6.2 ± 0.7 mL/2 h, p = 0.021), and no changes in 0.3 M NaCl (3.2 ± 0.8 vs sham 2.7 ± 0.5 mL/2 h, p = 0.842). The difference in the volume intake occurs in the first 30 minutes of observations, highlighted in the non-cumulative intake (Figure 1). Urinary volume and K+ excretion was not altered during Furo/Cap treatment. However, there was an increase in Na+ excretion (402.2 ± 30.1vs sham: 283.2 ± 23.1 mEq/L, p = 0.018, Figure 2) at the beginning of the measures.
Morphometric variables obtained by echocardiography in acute AR or sham rats.
Variables | AR (n = 9) | Sham (n = 8) | p value |
---|---|---|---|
LVDD (mm) | 7.30 ± 0.10* | 6.28 ± 0.20 | < 0.001 |
LVSD (mm) | 2.90 ± 0.30 | 2.50 ± 0.20 | 0.339 |
LA/Ao | 1.23 ± 0.10* | 1.03 ± 0.00 | 0.034 |
RWT | 0.40 ± 0.10 | 0.39 ± 0.00 | 0.702 |
LAA/RAA | 1.42 ± 0.10* | 1.23 ± 0.10 | 0.048 |
SI | 0.70 ± 0.00 | 0.61 ± 0.00 | 0.077 |
FS | 60.52 ± 3.80 | 59.69 ± 2.20 | 0.852 |
Cardiac output (mL/min) | 88.73 ± 9.70 | 64.99 ± 6.00 | 0.062 |
E (cm/s) | 74.22 ± 5.90 | 72.62 ± 4.00 | 0.830 |
A (cm/s) | 53.55 ± 5.70 | 43.75 ± 3.30 | 0.169 |
Values are means ± SEM. LVDD, left ventricle diastolic diameter; LVSD, left ventricle systolic diameter; LA/AO, left atrium systolic diameter normalized to aortic root diastolic diameter; RWT, relative posterior wall thickness; LAA/RAA, left atrium area normalized to right atrium area; SI, sphericity index of left ventricle; FS, fractional shortening of left ventricle; E and A, velocity waves of transmitral flow. One way analyses of variance followed by Student-Newman-Keuls test for comparisons were used to highlight significant differences.
Autonomic modulation following atropine and atenolol injections.
Parameters | AR (n = 5) | Sham (n = 5) | p value |
---|---|---|---|
SAP mmHg | 134.52 ± 5.77 | 137.30 ± 5.93 | 0.723 |
DAP mmHg | 66.28 ± 6.88* | 97.10 ± 3.22 | 0.05 |
MAP mmHg | 98.78 ± 5.27 | 115.00 ± 3.57 | 0.122 |
Vagal tonus (Δbpm) | 34.61 ± 4.39 | 37.38 ± 10.16 | 0.837 |
Sympathetic t. onus (Δbpm) | -28.20 ± 9.87 | -34.85 ± 9.89 | 0.700 |
Vagal effect (bpm) | -79.65 ± 9.97 | -65.26 ± 7.92 | 0.320 |
Sympathetic effect (bpm) | 25.82 ± 7.97 | 38.01 ± 6.88 | 0.291 |
HR | 329.86 ± 13.70* | 383.06 ± 11.86 | 0.05 |
IHR | 372.70 ± 5.32 | 401.31 ± 13.70 | 0.156 |
Body weight (g) | 307.55 ± 5.01 | 314.44 ± 9.60 | 0.535 |
Values are means ± SEM. SAP, systolic arterial pressure; DAP, diastolic arterial pressure: MAP, mean arterial pressure; HR, heart rate; IHR, intrinsic heart rate (following the blocked of muscarinic and adrenergic receptors in the heart). One way analyses of variance followed by Student-Newman-Keuls test for comparisons were used to highlight significant differences.
Hemodynamic measurements and autonomic modulation
The hemodynamic and autonomic modulations are shown in Table 2. The diastolic arterial pressure (DAP) was reduced in acute AR compared with that of sham (p = 0.05). The autonomic modulation was not different between groups (Table 2). The heart rate of the AR was lower than sham rats.
Heart rate variability and baroreflex sensitivity
The spectral analyzes of the pulse interval show no differences for very low frequency (VLF) and LF, but there was an increase in HF for rats with AR, p = 0.011 (Table 3). Analyzing the spontaneous BRS by the sequence method the acute AR rats present a decrease in the total gain compared with that of sham animals, p = 0.047 (Table 3).
Non-cumulative 0.3 M NaCl intake (above), water intake (below) and individual intake (right) in acute AR or sham rats challenged by combined Furo/Cap treatment. Results are expressed as means ± SEM, n = number of rats. One way analysis of variance followed by Student-Newman-Keuls, test showed a significant difference for water intake at the first 30 min (p = 0.027).
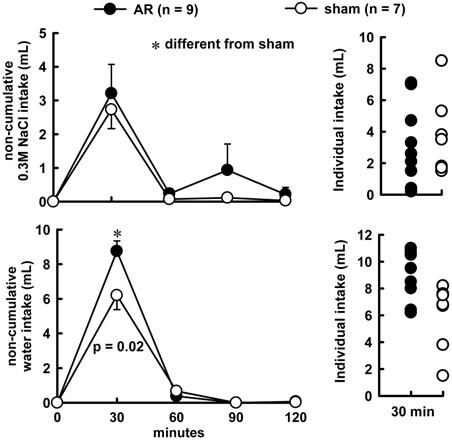
Urinary volume (left), sodium (middle) and potassium (right) excretion at the beginning of water and sodium intake (zero min) and at the end of observation (120 mim) in acute AR or sham rats. The animals received a combined Furo/Cap treatment one hour previous. One way analysis of variance followed by Student-Newman-Keuls test showed a significant difference for Na+ excretion at the zero min (p = 0.014).
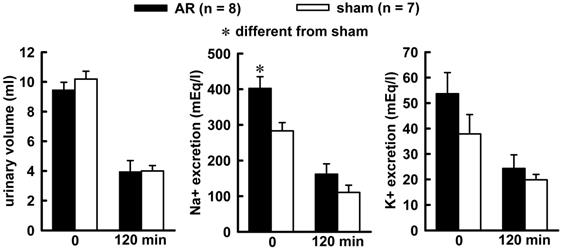
Heart rate variability and barorreflex sensitivity data.
Spectral analysis | AR (n = 6) | sham (n = 5) | p value |
---|---|---|---|
Systolic pressure | |||
VLF mmHg2 | 10.00 ± 2.11 | 9.09 ± 2.21 | 0.783 |
LF mmHg2 | 3.70 ± 0.72 | 3.96 ± 0.39 | 0.781 |
HF mmHg2 | 3.70 ± 0.52* | 1.70 ± 0.26 | 0.011 |
Pulse interval | |||
LH n.u. | 29.80 ± 3.57 | 28.40 ± 9.74 | 0.367 |
HF n.u. | 70.20 ± 3.57 | 71.60 ± 9.74 | 0.353 |
LF/HF | 0.70 ± 0.25 | 0.94 ± 0.68 | 0.401 |
Baroflex Gain | |||
up (ms/mmHg) | 1.13 ± 0.13 | 1.54 ± 0.15 | 0.153 |
down (ms/mmHg) | 1.08 ± 0.17 | 1.67 ± 0.27 | 0.697 |
all (ms/mmHg) | 1.10 ± 0.11* | 1.61 ± 0.17 | 0.047 |
Baroreflex index | 0.08 ± 0.02 | 0.10 ± 0.01 | 0.538 |
Values are means ± SEM. VLF, very low frequency; LF, low frequency; HF, high frequency. One way analyses of variance followed by Student-Newman-Keuls test for comparisons were used to highlight significant differences.
Discussion
The present results show that acute AR, which exposes the heart to abrupt volume overload, augmented water intake and natriureses following combined Furo/Cap treatment, and impaired the BRS. Our results showed reduced DAP, similar to what happens in AR patients. The echocardiograms results show an increased LVDD and the LA, either normalized by the Ao or per area, evidencing that the heart was exposed to volume overload. We investigated the fluid balance when only the heart is exposed to volume overload, but not other vital organs, e.g., kidneys or liver as can be seen in other studies10,14. This AR model is well tolerated by rats, and resembles to what happens in humans. The animals might be asymptomatic for a long period, almost 12 months following the induction7. As to the ECHO data the present results are in accordance with other studies that pointed out that LVDD starts to increase around 14 days of overloading8,9. The LVSD and fractional shortening is not altered by the AR in this acute phase maybe due to hypercontractility that tries to protect the heart from the increased volume.
The fluid homeostasis was disrupted by acute AR once water intake induced by Furo/Cap treatment was increased in the first 30 minutes of measuring; additionally, natriuresis in acute AR rats was observed. The AR produces an upregulation of extracellular matrix components, e.g., collagen and fibronectin7, which might cause impairment on the volume receptors of the heart. In dogs exposed to aorta cava fistule, damage on these cardiac receptors can be seen20. The combined treatment of Furo/Cap produces a hypotension in the animals, and this is essential for inducing water and sodium intake21. In an AR situation these receptors might lose the ability to adequately monitor circulating volume. As consequence the decrease in blood pressure produced by Furo/Cap treatment might be interpreted as more pronounced than in a sham situation. The circulatory system informs central sites that interpret it as the need to replace the fluid, what causes an increase in water intake. Regardless of sodium excretion, the natriuresis might be due to an increase in the circulating levels of the natriuretic peptides, such as atrial (ANP) or brain (BNP) natriuretic peptides. Two weeks after AR there was an increase in the expression of ANP and BNP genes in the LV7. It is well known that these natriuretic peptides are both secreted from the heart as a result of wall stress produced by volume or pressure to the heart, and also that they protect the body from volume overload11. In this way, the natriuresis observed might be, at least in part, a consequence of an increase in circulating ANP produced by the volume overload caused by AR. As to natriuresis, it is worth highlighting that volume expansion close to the heart determines a decrease in renal nerve activity10,14,15. Furthermore, the natriuresis in the acute AR might be the combination of increases in circulating ANP and decreases in renal nerve activity determined by the volume overload in the cardiac receptors close to the heart. It is well known that in chronic volume overload to the heart the fluid homeostasis is disrupted. It is worth noting that these data show that since from the earliest stages of volume overload to the heart the fluid balance is most likely to be disrupted, since the information of the cardiac volume receptors on the central sites controlling the intake behavior has already been injured.
The diastolic blood pressure (DAP) is low in AR rats, similar to what happens in humans3,5. The decrease in DAP may result from volume overload to the cardiac receptors, which respond with decreased vascular peripheral resistance when stimulated22. The present results show no differences in autonomic modulation to the heart in AR, both sympathetic and vagal flow to the heart were similar. The HRV analysis shows an increase in the HF component, which reflects the sympathetic outflow, in the AR rats. Meanwhile, it is worth considering that in the AR condition there are some physiological effects, e.g., arrhythmic events. Once the pharmacological challenge of autonomic tonus was not altered, the increase in HF component might reflect not an increase in the parasympathetic outflow to the heart, but instead of some arrhythmic event. On the other hand, there was a decrease in the BRS in the acute AR. The decrease in the BRS, similar to what happens to increases in water intake, might be impairment in the cardiac receptors in monitoring the volume overload in these rats. After two weeks of volume overload to the heart the baroreceptors got used to this high volume of blood. Our experiments were conducted during an acute phase of volume overload to the heart but similar results were found in chronic volume overload experiments. Although, dogs with heart failure present reduced sensitivity to arterial baroreceptors, they preserve the baroreceptor control of renal sympathetic nerve activity23. Measuring the renal sympathetic nerve activity was not our focus but we observed natriureses in the present study, which might reflect a similar condition. In conclusion, the results pointed that acute AR produces impairment to the fluid balance and decreases BRS, but it does not change autonomic modulation to the heart at the onset of volume overload to the heart.
Methods
Animal Model of AR
Male Wistar rats (260 to 280 g) had sham or AR surgeries induced by multiple retrograde puncture of the aortic valve leaflets under anesthesia (ketamine 80 mg/kg of bw plus xylazine 7mg/Kg bw, Vetbrands, Jacareí-SP) as described elsewhere24,25,26. All procedures were in accordance with the Animal Care and Use policies of the Institute of Biosciences of Botucatu, according to the Brazilian College of Animal Experimentation (COBEA, number 141/09) policies. Daily observations were made to minimize not only the number of animals used but also their discomfort.
Echocardiography
A complete M-mode, 2D, and Doppler echocardiogram was performed in the animals under anesthesia (ketamine 80mg/kg and xilazyne 20mg/kg) using an 11-Mhz probe of GE 6S echograph to confirm the presence and severity of AR at week 1. The criteria inclusion of the AR animals in the study was a ratio of regurgitate jet width to LV outflow diameter ≥ 50% of a retrograde holo-diastolic flow in the proximal descending aorta. Animals that failed to meet these criteria were excluded. We analysed LV dimensions, wall thickness, ejection fraction, diastolic function, and cardiac output (i.e., ejection volume in the LV outflow tract-heart rate), as previously reported24,25,26.
Drugs
Diuretic/natriuretic, furosemide (Furo, Pharmaceutical and Chemicals, Barbalha, Ceará, Brazil), 10 mg/kg of body weight, and angiotensin converting enzyme inhibitor, captopril (Cap, Captotec®, Hexal, Cambé, Paraná, Brazil), 5 mg/kg of body weight, were administered subcutaneously (sc). Atropine methyl nitrate, a muscarinic acetylcholine receptor antagonist (atropine, Sigma, USA), 3 mg/kg, and atenolol, a β-adrenergic blocker (Medley, Campinas, São Paulo, Brazil), 6 mg/kg, were injected intravenously.
Daily water and 0.3 M NaCl intake
The rats were kept in individual metal cages for one week for daily sodium and water intake measures. Water and 0.3 M NaCl were provided from burettes with 100 ml divisions that were fitted with metal drinking spouts, with free access to standard laboratory diet (Labina Purina® Rat Chow). Temperature was maintained at 23 ± 2º C and humidity at 54 ± 10%, on a 12:12 light:dark cycle (onset at 06:10). Overnight urine samples were collected at the end of the first week. Urine samples were analysed by a flame photometer to quantify Na+ and K+ in milliequivalents (mEq). In order to calculate sodium and potassium excretion the urinary volume was multiplied by the mEq.
Acute fluid depletion
Fluid depletion was induced by combining injections of Furo and Cap. This protocol induces sodium and water intake in a short time (1 h) due to hypotension and increases of circulating angiotensin I to be converted in angiotensin II in the central sites without blood brain barrier which induces fluid behaviour21,27. The rats were removed from their cages, injected with Furo/Cap, and returned to their cages from which water and saline had been removed. One h later, burettes of 0.3 M NaCl and water were returned and intakes of both were measured at 30, 60, 90 and 120 min. Urinary volumes were measured and a sample was collected at zero (0 min) and at 120 min for posterior analyses. The urine samples were kept in the freezer in order to have Na+ and K+ quantified by a flame photometer.
Hemodynamic autonomic modulation
On day 12 the rats were anesthetized again with ketamine (80 mg/kg of body weight) combined with xylazine (7 mg/kg of body weight) and a polyethylene tubing (PE-10 connected to a PE-50, Braintree Scientific, Inc. MA, USA) was inserted into the abdominal aorta through the femoral artery and another cathether was inserted in the jugular vein for drug injections. The catheters were tunneled sc and exposed on the back of the rat to allow access in unrestrained, freely moving rats in the following day. In the day of the experiments the arterial catheter was connected to a pressure transducer (TSD104A, Biopac Systems) coupled to a pre-amplifier (model M100A-CE, Biopac SystemsSanta Barbara, CA, USA) connected to a BIOPAC computer data acquisition system (sampling set as 2000 samples/second). A basal recording of 50 min of the pulsatile arterial pressure (AP), mean AP (MAP) and heart rate (HR) was carried out autonomic modulation tests. Autonomic influence to the heart was studied by injecting atropine (3 mg/kg, iv; Sigma) and atenolol (6 mg/kg, iv; Sigma) in a maximal volume of 0.2 ml per injection. In the first day of recordings, after the basal recording, atropine was injected, after ten minutes atenolol was injected. Then this double blocking provided the intrinsic heart rate (IHR, similar to 28). On the second day of recordings the sequence of injections was reverted, i.e. atenolol injections were followed by atropine injections. The average of the HR beats daily was used to calculate sympathetic and vagal tonus. Sympathetic tonus was determined by taking into consideration the highest HR after atropine minus the IHR. Vagal tonus was obtained by the difference between the lowest HR after atenolol injections and IHR.
Heart rate variability and spontaneous baroreflex sensitivity
The basal record was used to calculate the heart rate variability (HRV). The pulse interval was extracted from the systolic arterial pressure (SAP) in the time frequency domain. The beat-to-beat SAP and HR data were converted into data points every 100 ms using a cubic spline interpolation (similar to 29). We used the free software CardioSeries v2.4 (www.danielpenteado.com) to assess the frequency domain by means of autoregressive spectral analysis. The oscillatory components were quantified in low (LF: 0.2 to 075 Hz) and high frequency (HF: 0.75 to 3.0 Hz) ranges. Using the same program we calculated the baroreflex sensitivity according to the sequence method.
Data analysis
Data are presented as mean ± standard error. T-test was used to compare echocardiograms variables, fluid intake, overnight urinary output, hemodynamic measurements, autonomic modulation, HRV and BRS. One-way ANOVA analyses of variance followed by Student-Newman-Keuls test were used to compare groups.
Acknowledgements
This study was supported by São Paulo Research Foundation - FAPESP process # 2009/52547-8.
Competing Interests
The authors have declared that no competing interest exists.
References
1. Bonow RO, Cheitlin MD, Crawford MH, Douglas PS. Task Force 3: valvular heart disease. J Am Coll Cardiol. 2005;45(8):1334-40
2. Carabello BA, Crawford FA Jr. Valvular heart disease. N Engl J Med. 1997;337(1):32-41
3. Nishimura RA, Otto CM, Bonow RO, Carabello BA, Erwin JP 3rd, Fleisher LA, Jneid H, Mack MJ, McLeod CJ, O'Gara PT, Rigolin VH, Sundt TM 3rd, Thompson A. AHA/ACC Focused Update of the 2014 AHA/ACC Guideline for the Management of Patients With Valvular Heart Disease: A Report of the American College of Cardiology/American Heart Association Task Force on Clinical Practice Guidelines. J Am Coll Cardiol. 2017;70(2):252-289
4. Gustavsson CG, Gustafson A, Albrechtsson U, Larusdottir H, Stahl E, Olin C. Diagnosis and management of acute aortic dissection, clinical and radiological follow-up. Acta Med Scand. 1988;223:247-253
5. Mann T, McLaurin L, Grossman W, Craige E. Assessing the hemodynamic severity of acute aortic regurgitation due to infective endocarditis. N Engl J Med. 1975;293:108-113
6. Borer JS, Truter SL, Gupta A, Herrold EM, Carter JN, Lee E, Pitlor L. Heart failure in aortic regurgitation: the role of primary fibrosis and its cellular and molecular pathophysiology. Adv Cardiol. 2004;41:16-24
7. Champetier S, Bojmehrani A, Beaudoin J, Lachance D, Plante E, Roussel E, Couet J, Arsenault M. Gene profiling of left ventricle eccentric hypertrophy in aortic regurgitation in rats: rationale for targeting the beta-adrenergic and renin-angiotensin systems. Am J Physiol Heart Circ Physiol. 2009;296(3):H669-77
8. Lachance D, Plante E, Roussel E, Drolet MC, Couet J, Arsenault M. Early left ventricular remodeling in acute severe aortic regurgitation: insights from an animal model. J Heart Valve Dis. 2008;17(3):300-8
9. Roussel E, Gaudreau M, Plante E, Drolet MC, Breault C, Couet J, Arsenault M. Early responses of the left ventricle to pressure overload in Wistar rats. Life Sci. 2008;82(5-6):265-72
10. Badoer E, Moguilevski V, McGrath BP. Cardiac afferents play the dominant role in renal nerve inhibition elicited by volume expansion in the rabbit. Am J Physiol. 1998;274(2 Pt 2):R383-8
11. de Bold AJ, Ma KK, Zhang Y, de Bold ML, Bensimon M, Khoshbaten A. The physiological and pathophysiological modulation of the endocrine function of the heart. Can J Physiol Pharmacol. 2001;79(8):705-14
12. Kaufman S. Role of right atrial receptors in the control of drinking in the rat. J Physiol. 1984;349:389-96
13. Toth E, Stelfox J, Kaufman S. Cardiac control of salt appetite. Am J Physiol. 1987;252(5 Pt 2):R925-9
14. Colombari DS, Colombari E, Lopes OU, Cravo SL. Afferent pathways in cardiovascular adjustments induced by volume expansion in anesthetized rats. Am J Physiol Regul Integr Comp Physiol. 2000;279(3):R884-90
15. Thames MD, Miller BD, Abboud FM. Baroreflex regulation of renal nerve activity during volume expansion. Am. J. Physiol. 1982:243 H810-H814
16. Berntson GG1, Bigger JT Jr, Eckberg DL, Grossman P, Kaufmann PG, Malik M, Nagaraja HN, Porges SW, Saul JP, Stone PH, van der Molen MW. Heart rate variability: origins, methods, and interpretive caveats. Psychophysiology. 1997;34(6):623-48
17. Malliani A, Pagani M, Lombardi F, Cerutti S. Cardiovascular neural regulation explored in the frequency domain. Circulation. 1991;84(2):482-92
18. Parati G, Di Rienzo M, Mancia G. How to measure baroreflex sensitivity: from the cardiovascular laboratory to daily life. J Hypertens. 2000;18(1):7-19
19. Pinna GD, Maestri R, La Rovere MT. Assessment of baroreflex sensitivity from spontaneous oscillations of blood pressure and heart rate: proven clinical value? Physiol Meas. 2015;36(4):741-53
20. Zucker IH, Earle AM, Gilmore JP. Changes in the sensitivity of left atrial receptors following reversal of heart failure. American Journal of Physiology. 1979:237 H555-H559
21. Thunhorst RL, Johnson AK. Renin-angiotensin, arterial blood pressure, and salt appetite in rats. Am J Physiol. 1994;266(2 Pt 2):R458-65
22. Ludbrook J, Graham WF. The role of cardiac receptor and arterial baroreceptor reflexes in control of the circulation during acute change of blood volume in the conscious rabbit. Circ Res. 1984;54(4):424-35
23. Dibner-Dunlap ME, Thames MD. Reflex control renal sympathetic activity is preserved in heart failure despite reduced arterial baroreceptor sensitivity. Cir Res. 1989;65:1526-1535
24. De Gobbi JI, Omoto AC, Siqueira TF, Matsubara LS, Roscani MG, Matsubara BB. Antidepressant treatment decreases daily salt intake and prevents heart dysfunction following subchronic aortic regurgitation in rats. Physiol Behav. 2015:15 144:124-8
25. Roscani MG, Polegato BF, Minamoto SE, Lousada AP, Minicucci M, Azevedo P, Matsubara LS, Matsubara BB. Left ventricular sphericity index predicts systolic dysfunction in rats with experimental aortic regurgitation. J Appl Physiol (1985). 2014;116(10):1259-62
26. Omoto ACM, Moraes LN, Garcia GJF, Vechetti-Júnior IJ, Roscani MG, Carvalho RF, De Gobbi JIF. Paroxetine alters cardiac stress markers in rats with aortic regurgitation. (unpublished, submitted).
27. Fitts DA, Masson DB. Forebrain sites of action for drinking and salt appetite to angiotensin or captopril. Behav Neurosci. 1989;103(4):865-72
28. De Angelis KL, Oliveira AR, Dall'Ago P, Peixoto LR, Gadonski G, Lacchini S, Fernandes TG, Irigoyen MC. Effects of exercise training on autonomic and myocardial dysfunction in streptozotocin-diabetic rats. Braz J Med Biol Res. 2000;33(6):635-41
29. Fazan R Jr, Dias da Silva VJ, Ballejo G, Salgado HC. Power spectra of arterial pressure and heart rate in streptozotocin-induced diabetes in rats. J Hypertens. 1999(174):489-95
Author contact
Corresponding author: Juliana Irani Fratucci De Gobbi, Department of Physiology, Institute of Bioscience, UNESP, São Paulo State University, UNESP, Distrito de Rubião Junior s/n, 18618-000 Botucatu- SP, Brazil. Phone: +55 (14) 3880-0319, -0330. Fax: +55 (14) 3815-3744; Email: juliana.gobbibr, degobbijulianacom