J Biomed 2019; 4:14-34. doi:10.7150/jbm.34611 This volume Cite
Review
Exosomes: Biological Couriers with Transformative Messages
1. Biotechnology Graduate Program, School of Sciences and Engineering, the American University in Cairo
2. Institute of Global Health and Human Ecology, School of Sciences and Engineering, the American University in Cairo.
Abstract
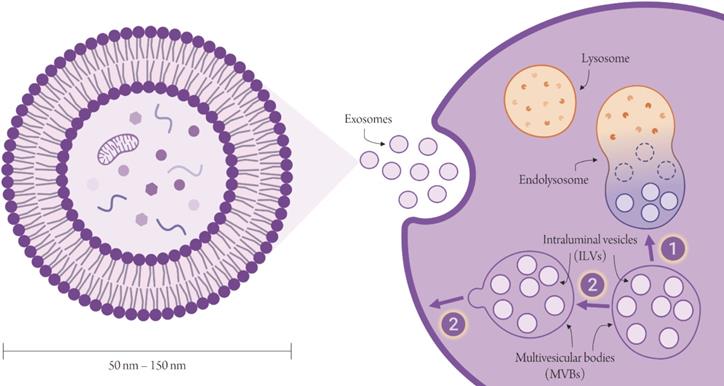
Exosomes are nanovesicles produced by almost all cell types to the extracellular environment. These structures, initially mistaken for “cellular dust”, are key effectors of cell-cell communication and play major roles in maintaining normal physiological function and homeostatic balance as well as relaying pathology. Given their non-immunogenicity, their native membrane composition combined with the wealth of contained bioinfomative cargoes and coupled to their innate ability to cross multiple biological barriers, these tiny sacs have merited a great deal of interest in recent years and are slowly edging their way to the surface. Accruing evidence corroborating the far-reaching potential of exosomes is ever growing and easily demonstrable. Nonetheless, progress with translation of the reported outcomes to clinical settings and recognition of these tools as a multi-application platform remains much too inadequate for the outstanding potential of these biological messengers and the entailed shift in theragnostic paradigms. The purpose of this review is to recapitulate on the most recent advances in exosome research and accentuate their momentous promise as game changers in disease diagnostics and therapeutics. Finally, market transition and commercialization efforts are highlighted with a brief commentary on the status quo.
Keywords: Exosomes, Extracellular Vesicles, EVs, Biomarkers, Theragnostic
1. “E” is for Exosomes
Exosomes are the smallest type of extracellular vesicles (EVs) with sizes ranging between 50 - 150 nm [1]. Unlike the larger microvesicles, exosomes are not produced by mere shedding of the plasma membrane, but are the product of a more complex biogenetic pathway. Exosomes begin as intraluminal vesicles (ILVs) within - as their name suggests - the lumen of late endosomes, also known as multivesicular bodies (MVBs). Of the two possible fates of MVBs, only one eventually culminates in the release of exosomes at the cell surface [2,3] (Figure 1). MVBs that escape degradation by lysosomes migrate to the cell surface. On fusion of their endosomal limiting membrane with the plasma membrane, MVBs release their load of ILVs, now properly called exosomes to the extracellular space. These exosomes exhibit surface proteins that are unique to these specific subcellular structures and are primary targets for exosome separation. They also carry cargos of biomolecules that - being collected from the cytosol of the donor cell - are essentially a sample of their cells of origin and are capable of relaying a wealth of information “sent” by these cells to other body sites. A number of data repositories such as exoRBase, ExoCarta, Vesiclepedia and EVpedia, carry a compendium of all EV-related knowledge, including identified EV-freighted cargos and EV-specific markers in addition to comprehensive compilations of EV-based studies and publications. (Figure 2) shows statistics pertaining to the abovementioned databases.
Many naming conventions have been used in the literature to refer to extracellular vesicles and their subtypes [8] (Table 1). Owing to a degree of overlap across the EV subtypes in regards to size and a few structural features, effective separation has not been fully achieved [2]. As such, in many instances, authors do not apply appropriate terminology to refer to the EVs their work involved; where some studies describe their EVs as exosomes (based on a set of exosome-specific markers and a size range conformity), others prefer the generic term of “extracellular vesicles”. Such discrepancies complicate the interpretation of reported outcomes, despite a technical accuracy with terminology usage. A broad naming consensus does however exist and is satisfactorily reliable. The currently standard nominal categorization recognizes two groups of extracellular vesicles: exosomes and microvesicles. A third marginally relevant group is comprised of apoptotic bodies. True to their namesake, these are fundamentally fragments of apoptotic cells, produced in the course of programmed cell death. They are, however, biochemically and morphologically more similar to microvesicles than exosomes.
Ideally, studies should report the characteristics of the isolated EVs, which would hugely simplify the ascription of study outcomes to a specific EV subgroup. Notwithstanding the above, exosomes have been brought in the limelight, ahead of other EVs, most likely due to their nanoscale dimensions, which allows them to deliver messages remotely and across various biological barriers. On that account, this article will focus on exosomes over microvesicles.
2. Biogenesis
Biogenesis of all EV types involves membrane trafficking pathways typical of vesicular production. EV cargo sorting, vesicular formation and release can occur in a canonical ESCRT-dependent and non-canonical ESCRT-independent pathways [9]. Exosome biogenesis is an extremely complex biological process, involving numerous interconnected pathways and has been thoroughly studied and described each in isolation. Given that a circumstantial description of exosome biogenesis lies beyond the scope of this review and its purposes, only a compendious briefing of the well-understood mechanisms is provided with a closer look on the most recently uncovered mechanisms.
Various EV naming conventions. Extracellular vesicles have been classified according to their tissue of origin; “dexosomes”: EVs from intestinal epithelial cells; “oncosomes”: EVs from cancer cells; “prostasomes”: EVs from prostate epithelium and “prominsosomes”: EVs from neural stem cells. EVs have been also named according to the function they perform; “tolerosomes”: EVs imparting tolerance ingested antigenic components; “migrasomes”: EVs mediating cellular migration; “reprosomes”: EVs moderating reprogramming of differentiated cells to other niches. Terminologies with reference to EV size have been coined as well.
Donor cells | Function | Size | Extracellular presence | Other |
---|---|---|---|---|
Dexosomes Oncosomes Prostasomes Prominosomes | Tolerosomes Migrasomes Reprosomes | Nanovesicles Microvesicles | Exosomes Ectosomes Exovesicles | Shedding vesicles Blebbing vesicles |
Biogenesis of exosomes. Exosomes are produced in the course of the endocytic pathway. MVBs (containing ILVs) have either one of two fates; either they fuse with lysosomes to form the endolysosome, in which all components are degraded, or they migrate to the surface of the cell and have the endosomal limiting membrane fuse with the plasma membrane, releasing ILVs aka exosomes into the extracellular environment. Created with Biorender.com.
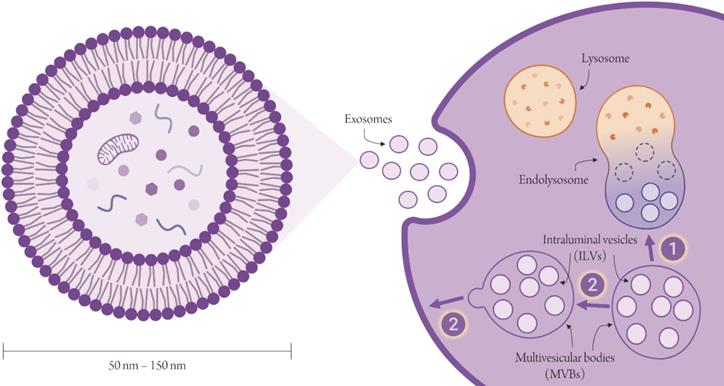
EV-related digital compendia and databases. The outlined statistics reflect the present content. Number of entries do not concur with number of unique molecules due to duplications. The number of total EVpedia publications is 14,192 as of April 30th, 2018. The number of EVpedia-carried publications shown in the figure reflects solely the number of eukaryotic-pertaining studies and reflects the latest update released by EVpedia.
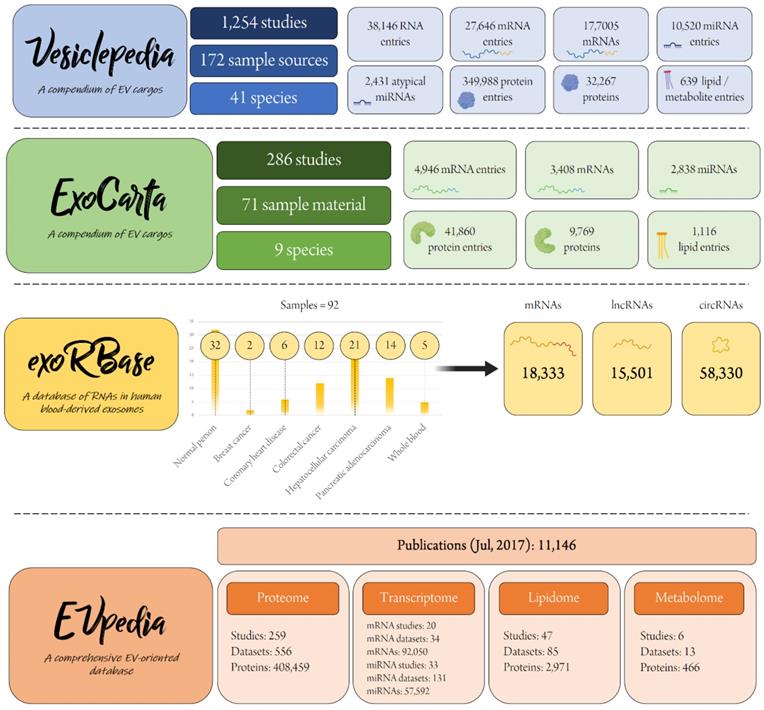
ESCRT accessory proteins.
Protein | Name | Interaction | Function | Ref. |
---|---|---|---|---|
ALIX | ALG2 interacting protein X; Programmed cell death 6interacting protein | ESCRT-I and ESCRT-III | Linking ESCRT-I and ESCRT-III; participate in membrane budding | [14] |
ARRDC1 | Arrestin Domain Containing 1 | ALIX and TSG101 (subunit of ESCRT-I) | Membrane budding | [15] |
DID2 | DOA4-independent degradation protein 2 | ESCRT-III and Vps4 | Bridging vps4 and ESCRT-III | [16] |
IST1 | Increased sodium tolerance 1 | Vps4, LIP5, DID2 and ESCRT-I | Participates in disassembly of Vps4 | [17] |
CHMP5/Vps60 | Vacuolar protein sorting-associated protein 60 | LIP5 | Vps4 stimulation | [18] |
2.1. ESCRT-dependent pathway
The implication of the endosomal sorting complex required for transport (ESCRT) in formation of ILVs within MVBs has been a well-characterized mechanism of exosome biogenesis. ESCRT is a family of 4 protein complexes (ESCRT-0, ESCRT-I, ESCRT-II and ESCRT-III) (Figure 3) and, together with their accessory proteins (Table 2) moderate various steps of the biogenesis cascade [3,10].
A breakdown of the ESCRT machinery, showing the constituent subunits of each ESCRT complex and delineating the sequential functionality of each member of the machinery. Complex subunits (left) are shown in humans in the same order given for their yeast counterparts; subunits involved in mechanistic interactions are bolded and italicized. Created with Biorender.com.
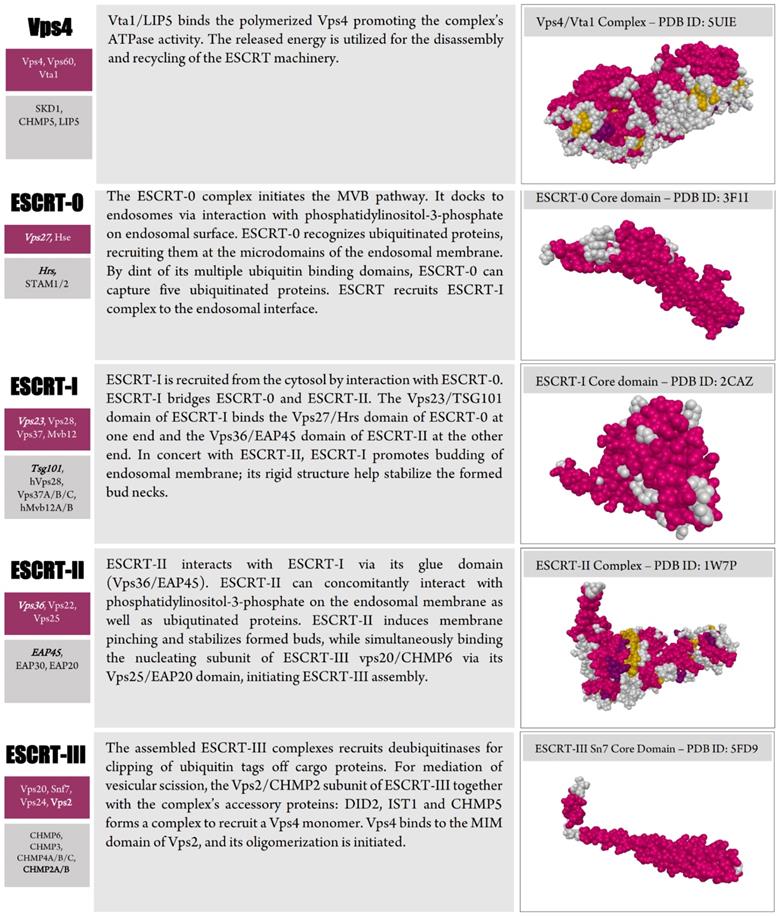
ESCRT disruption has been associated with changes in cargo composition [3], which indicates its involvement in cargo sorting. ESCRT-0 recruits ubiquitinated proteins to microdomains on the endosomal limiting membrane, while ESCRT-I and -II modulate membrane remodeling into outgrowths with the rounded up cargo. Eventually, ESCRT-III moderates vesicular scission. ESCRT-associated proteins, namely Vps4 ATPase drive the disengagement and recycling of the ESCRT machinery [3]. A study published in Biophysical Journal [11] reported that inhibition of the ESCRT-III-associated AAA+ ATPase Vps4 in HEK293 cells depletes protein and miRNA cargoes of EVs with a downturn in EV release. Vps4 inhibition was shown to negatively affect biogenesis and release of exosomes and microvesicles alike, which is evinced by a decrease in CD63 and CD9 tetraspanins1 (putative exosomal markers); such a universal decline likely reflects the role of ESCRT-III in biogenesis of the various EV subpopulations, rather than exclusively exosomes. Rather than an associated protein, Vps4 has been classified, together with its Vta1 cofactor, by researchers in the field as a fifth component of the ESCRT complex [12]. ESCRT components have also been shown to conduce to nucleic acid loading. For example, ESCRT-II subcomplex have been postulated to act as an RNA-binding complex, which shuttles microRNAs into the forming vesicles in a selective manner, given a protein-RNA sequence complementarity. Such RNA-bound proteins can then be recruited to specific membrane microdomains enriched in tetraspanins (components of ESCRT-independent biogenesis mechanisms), discussed below [13].
2.2. ESCRT-independent mechanisms
Various efforts have unraveled a number of non-canonical pathways of exosome biogenesis. Ceramide production by neutral sphingomyelinase-mediated hydrolysis of sphingomyelin is a primary ESCRT-independent mode of exosome biogenesis, whereby ceramide engenders the inward curvature of the endosomal membrane through formation of subdomains that promote invagination of the lipid bilayer [19]. Neutral sphingomyelinase-mediated biosynthesis of ceramide was reported to be requisite for exosomal secretion of microRNAs [20]. Moreover, suppression of neutral sphingomyelinase diminished the importation of lipoproteins in exosomes, whereas its upregulation promoted vesicular budding from lipid rafts[21] - lipid ordered membrane, detergent-resistant domains enriched in sphingolipids, cholesterol and GPI2-anchored proteins. Lipid rafts have been known for their involvement in endocytosis and vesiculation by virtue of their divergent biophysical properties and have been identified in exosomal membranes[22]. Drawing on this observation, lipid raft-associated cargos are - by extrapolation - anticipated to be recruited into early endosomes and subsequently enriched in exosomes, a hypothesis that has been validated on multiple occasions [22,23,24,25].
Another central mediator of ESCRT-independent exosome biogenesis is the tetraspanin protein family. CD63, CD9 and CD81 are distinctively prolific in exosomal membranes and have long been established exosomal markers; these transmembrane proteins effect a pivotal part in trafficking cargos into exosomes [26]. Tetraspanins assemble together or with other membrane or cytosolic proteins into microdomains [27]- usually abundant in lipid rafts (see above) - which kickstarts vesicular budding.
Adiponectin, an adipokine exclusively produced in adipose tissue and abundantly found in serum of healthy individuals, has recently been reported to stimulate exosome production together with a GPI-anchored cadherin protein, T-cadherin [28]. Based on several studies corroborating the subcellular presence of GPI-anchored proteins and their accumulation in MVBs, the authors hypothesized that adiponectin incorporates into the endocytic pathway via T-cadherin. They showed that adiponectin and ceramide co-accumulate with T-cadherin in MVBs of T-cadherin-expressing endothelial cells, producing adiponectin/T-cadherin-freighted exosomes in response to adiponectin. Total exosome production was found to be improved in vitro by the syndecan/syntenin system3. Moreover, the adiponectin stimulus encouraged packaging of ceramides into the forming MVBs, keeping its cellular levels in check. The adiponectin/T-cadherin-mediated biogenesis and release of ceramide-rich exosomes plays a crucial and direct role in the maintenance of cellular sphingolipid homeostasis and provides an explanation for the organ protective effects long attributed to the adiponectin/T-cadherin system. Another purported key regulator of late endosomal and MVB trafficking is the actin-nucleation-promoting factor, cortactin. Two research groups [29,30] recently delineated a role for cortactin4 in exosome biogenesis. Overexpression and downregulation of cortactin in head and neck squamous cell carcinoma cells correlated with a commensurate variation of exosome secretion with no apparent alteration of exosomal cargo. Moreover, cortactin was demonstarted to occassion and stabilize MVB docking to the cell membrane at actin-enriched invadopodia5, for subsequent exocytosis. Cortactin binds the Arp2/3 complex at its N-terminus for nucleation and branching, while binding other membrane trafficking and cytoskeletal proteins at its C-terminus; such interaction stabilizes against actin network debranching. Coordinated regulation of actin dynamics is achieved by the interaction of the cortactin/Rab27a/coronin1b triade. Coronin1b antagonizes cortactin by binding actin at MVB-docking invadopodial sites promoting the destabilization of the actin filaments; such antagonism is opposed by Rab27a6, which sequesters coronin1b. Therefore, the interplay among these factors is essential for controlled docking of MVB to the plasma membrane.
In many instances, post-translational modification of proteins deflects their trajectory to MVBs and is the key driving force for targeting protein cargoes into exosomes. Of the various posttranslational modification patterns, ubiquitin and ubiquitin-like taggers are the most explored. Ageta et al. [31] described a ubiquitin-dependent post-translational modification (PTM) system, UBL/MUB, that regulates sorting of proteins into MVBs. Ubiquitin-like 3/membrane-anchored Ub-fold (UBL3/MUB) is a localized membrane protein that employs C-terminal cysteine residues to modify target proteins by formation of disulfide linkages, unlike conventional ubiquitin and ubiquitin-like modification, which involves covalent bonding between C-terminal glycine of ubiquitin and lysine of target proteins. In their experiments, UBL3 knockout mice showed a 60% decrease of protein levels in exosomes compared to the wild-type group. They demonstrated significant colocalization of transfected EGFP-UBL3 with endogenous CD63, a well-established exosomal marker, supporting the thesis that UBL3 is enriched in MVBs. Furthermore, 1241 proteins were found to interact with UBL3 at the C-terminal cysteine residues, 29% of which were identifiable as exosomal proteins. The group communicated that exogenous proteins can be UBL3-tagged for therapeutic purposes. They also suggest that inhibition of UBL3 protein tagging is a potentially promising strategy for disorders in which pathological proteins spread via exosomes.
3. Exosomes: allies or enemies?
The primordial import of exosomes lies in their mediation of intercellular communication in multicellular organisms in a paracrine and/or endocrine manner [2,8]. Therefore, exosomes in and of themselves are not of inherent physiologically beneficial or deleterious disposition, rather, they naïvely deliver the messages they have been freighted with. Whether the messages are of benign or malicious nature is a function of the donor cell.
As such, exosomes released from cancer cells are shipped off with pro-oncogenic cargos, whereas others produced in macrophages have immunomodulatory effects. A subset of exosomes isolated from bronchoalveolar lavage fluid (BALF), derived from the pro-inflammatory myeloid-derived regulatory cells (MDRCs) were shown to contain mitochondria as well as mitochondrial DNA and were traced to recipient T cells in vitro [32]. What is interesting is the fact that MDRCs are well-known regulators of T cell-mediated immune response in asthma, suggesting a role of exosomes as effectors of defense mechanisms. Similarly, a surge in nasal mucosa-derived exosomes (NMDEs) was reported on exposure to bacterial endotoxin [33], which was associated with a two-fold increase in expression of NOS and TLR4. These LPS-stimulated exosomes exhibited a considerable antimicrobial activity against Pseudomonas aeruginosa. Cells in receipt of NMDEs demonstrated a four orders of magnitude greater NO production, attributed to conjectured cargo transfer. An analysis of the NMDE proteome revealed 44 proteins related to innate immune function.
Contrariwise, exosomes can be pernicious biostructures when hijacked by different infective agents and consequently directed to spread the infection. This manipulative mechanism has been reported for HIV [34], EBV [35], HCV [36], as well as bacterial pathogens, specifically Mycobacterium tuberculosis [28,37]. Recently, a similar mechanism has been uncovered for exosomal-mediated transmission of flavivirus RNAs and proteins to humans from arthropods such as the Ixodes scapularis7 ticks [38]. The Langat virus (LGTV) is suggested to hijack the host tick exosomal system; the transformed exosomes - now particles of high infectivity - delivers the viral cargo to human cells. RT-qPCR confirmed the existence of viral RNA inside exosomes isolated from tick cells. Viral envelope proteins were also detected in these exosomes. Such exosomes successfully infected human keratinocytes (HaCaT cells). Exosomes from infected blood-brain barrier (BBB)-simulating bENd3 microendothelial cells similarly transmitted the infection to mouse N2a neuronal cells. Given its genomic homology to LGTV, similar results are expected with the encephalitis virus (TBEV). Despite their naïve nature, exosomes hijacked by infectious agents in such manner, passively provide stealth from the host's defense mechanisms; hence, their threat paradoxically lies in their lacking immunogenicity.
The duality of exosomal role is also ostensible within the complexity of the nervous system. Whereas oligodendroglial exosomes contribute to axonal protection in response to stress [39]; those regurgitated by microglia that have engulfed misfolded neurotoxic proteins such as Aβ oligomers, contribute to the pathology propagation, which is ironic, considering their source [40,41].
An abundance of evidence suggests that exosomes are major mediators of cancer dissemination and are at the heart of multiple oncogenic mechanisms that constitute the hallmarks of malignancy [42,43]. Orchestrating these effects through borne messages shipped off at originating neoplasms, these exosomes can be utilized as biomarkers of disease [44] and have been endlessly employed for therapeutic strategies [45].
4. Designer Exosomes
Considering their active biological role, constitutional alteration of exosomes has burgeoned into an intriguing research arena. Indeed, numerous studies involving exosome engineering have proven that these membranous structures are highly amenable to modification and that the chimeric end product of such manipulation can be of shattering biological moment [46]. With simple intervention, exosomes can be engineered to serve in three major applications (Figure 4): tumor vaccines, disease biomarkers (diagnostics), delivery systems (therapeutics).
There are two overarching modi operandi for engineering exosomes, viz. exogenous and endogenous modification (Figure 4C, 4D); exogenous methods entail isolating exosomes and engineering them in vitro before they are returned to the patient. Endogenous methods on the other hand rely on genetically engineering donor cells to constitutively produce the modified exosomes. As will be demonstrated, both approaches are widely utilized and have been applied with a variety of adjustments. Herein, brief exemplars of general strategies for exosome modification are exposited.
4.1. siRNA loading
Optimization of exosomal loading with desired cargos or orchestrating enhanced sorting of endogenous cargos constitutes a major investigational front. Stationing of siRNA cargos to exosomal membrane for modulation of gene expression to therapeutic outcomes has been shown to significantly improve upon conjugation to a lipid moiety such as cholesterol. Variation of the lipid linker was found to correlate with exosomal association. A particularly prominent group in this area, showed a positive correlation between the hydrophobicity of the lipid and the loading efficiency [47,48]. This packaging approach was implemented to loading Huntingtin gene-silencing siRNAs onto mesenchymal stem cells (MSCs)-derived exosomes. The group also optimized the silencing activity of the RNA by using several backbone, sugar and 5'-end modifications for protection of the lipid-conjugated RNA against degradation by RNases. Several chemically-modified variants of the cholesterol-linker-siRNA conjugate were tested for their stability and silencing capacities. The highest silencing activity was reported for a 5'-(E)-vinylphosphonate-conjugated species, which further ameliorated on 2-fluoro and 2-O-methyl alternating modifications of all ribose moieties. 5-(E)-vinylphosphonate unassisted backbone alteration was conversely inconsequential. On that account, siRNA phosphatase sensitivity was suggested to be of greater significance in EV-mediated siRNA delivery than nuclease sensitivity, contrary to free siRNAs, for which nuclease-resistance is substantially critical.
To obviate endosomal sequestration of the siRNA and permit its necessary interaction with the RNA-induced silencing complex (RISC) in the cytoplasm, cleavable linkers are recommended for optimal knockdowns. Linker disruption eliminated the silencing capacity of the exosomes, reflecting unsuccessful conjugation in absence of a stable linker and failure of siRNA capture. Moreover, exosome functionality was subject to saturation kinetics, with siRNA overloading significantly compromising the silencing activity, owing to potential agglomeration and ensuing disruption of the lipid bilayer. A quota of around 3,000 siRNA copies per exosome is suggested, although such a number would vary with disparate hydrophobic conjugates and is a function of the nature of the exosomal membrane, which is largely dependent on the cell of origin.
A schematic outlining the most prominent modes exosome theranostics. A. Exosomal vaccines can be developed as a modality of cancer immunotherapy. Tumor cell-derived exosomes are isolated from peripheral tissue (most commonly plasma or serum), as well as patient's immune cell precursors, which are cultured to matured dendritic cells. The exosomes (freighted with oncogenic cargo) are then electroporated into the matured dendritic cells and these are administered to the patient, where they initiate an immune response by T lymphocytes that recognize the expressed oncogenic protein on the surface of the transformed dendritic cells; B. Exosomes (released by cells within the diseased tissue) can be isolated from various body fluids as a liquid biopsy biomarker; C. Exogenous drug loading into exosomes is carried out by electroporating the drug into isolated exosomes, which are then returned to the patient; D. Endogenous drug loading entails transfection of donor cells with a plasmid encoding the biomolecule of interest. The transfected cell produce modified exosomes, which can be isolated and administered to patients. Created with Biorender.com.
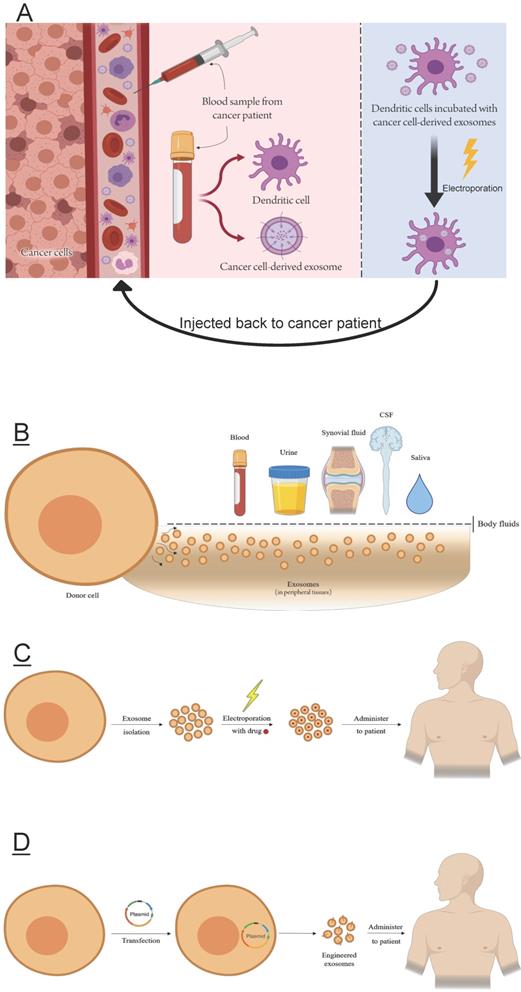
This approach is transferable to other RNA species and further investigation into its applicability in challenging delivery issues is encouraged, given the fact that a significant proportion of therapeutic strategies rely on gene silencing via siRNA cargos.
Transfection of exogenous RNAs into exosomes can otherwise be achieved by sonication, electroporation, calcium chloride and lipofection [21].
4.2. Protein loading
As highlighted above, sorting of exosomal protein cargos is mostly driven by ubiquitination of these proteins, which marks these proteins for recruitment to the endosomal membrane microdomains and renders them recognizable by the ESCRT machinery. Based on current understanding of exosomal loading mechanisms, researchers seek to modify biologically active proteins for recognition by the relevant machinery and consequent packaging into exosomes. Cheng and Schorey [49] devised an approach whereby a C-terminal ubiquitin tag targets soluble proteins to exosomes. This tactic was applied to test the loading efficiency of the ubiqutinated Mycobacterium tuberculosis Ag85B-ESAT6-Ub fusion protein into HEK293 exosomes, with a view of developing an exosome-based vaccine. HEK293 cells were engineered to co-express the two fused proteins, either with the ubiquitin tag or without. Exosomes from both transformants carried cargos with the fusion protein. However, exosomes from transformants with the ubiquitin tag harbored the protein in amounts 10 orders of magnitude compared to the ubiquitin-free group. Upon intranasal immunization of C57BL/6 mice, the exosomal concentration of fusion protein correlated with an upsurge of specific IFNγ-secreting T lymphocytes.
In a proof of concept study, Sterzenbach et al. [50] exploited several aspects of the endocytic mechanism for loading MVBs and successfully engineered exosomes to deliver Cre recombinase8 into murine brains. They demonstrated that flagging Cre recombinase (protein of interest) with a specific tag, renders it recognizable by the L-domain of Ndfip1 protein9, which in turn marks the protein for ubiquitination (by Nedd4 ubiquitin ligases) and ESCRT-mediated loading into exosomes. The ability of these exosomes to effect functional delivery of the enzyme was validated by their competence to mediate DNA recombination in floxed reporter cells. The study further explored the capacity of these exosomes to trigger DNA recombination events in the brains of transgenic floxed mice. The exosomes, administered via the intranasal route, were detected in various brain regions. The study supports the conviction that exosomes can be excellent tools for delivery of therapeutic agents across the blood brain barrier and presents a model system for further experimentation.
4.3. Surface display
Beyond integrating disease-modifying agents and loading enhancers, exosomes can be structurally altered for sundry purposes. Incorporation of a targeting moiety into the exosomal membrane is by far the most commonly applied engineering strategy. Surface display of a targeting peptide may be - in many instances - sufficient to bring about the desired outcome. Studies seeking the amelioration of exosome targeting are almost as abound. For example, glycosylation of the targeting peptide was shown to effect stabilization against proteolytic degradation[51]. Furthermore, a sizable body of research is dedicated to exploring modifications where exosomes bearing a surface tag or internalizing a labeled cargo can be traced for imaging or assessment of drug kinetics among other applications.
By way of illustration, a surface modification method proposed by Wan et al.[52] hinges on in situ nanoassembly of DNA aptamers on exosomal surface markers. Their aptamer - designed to recognize surface molecules on HepG2 cells-derived exosomes - constituted a seed in a hybridization chain reaction. The proposed nanoassembly is achieved when the aptamer recognizes its target molecule. Subsequent addition of two hairpin monomer species with complementary sequences initiates iterative polymerization, only triggered in presence of the exosome-bound aptamer. Polymerization thus occurs selectively on the surface of target exosomes. FITC tags can functionalize the monomeric units of assembled polymers to the overall effect of a 10-fold amplified signal compared to a simple apatmer-target conjugate; such a strategy represents exogenous modification of exosomes.
Inasmuch as aptamers have been used for exosome modification for diagnostic purposes, they can be an intriguing targeting modality for therapeutics. Exosomes were rendered nucleolin-targeting by vectorization with lipopeptide-aptameric constructs [36]. The aptamers were attached to the exosomal membrane via a cholesterol-peptide anchor. These exosomes efficiently homed to breast cancer cells and mediated therapeutic gene knockdown via delivery of let-7 miRNA.
While the above represent the exogenous approach to engineering or manipulating exosomes, tagging exosomes can alternatively be acquired by surface modification of donor cells (endogenous modification). In a recent work, Alberti and colleagues [20] implemented metabolic glycoengineering of exosomes-secreting cells, where MSCs were incubated with a modified sugar, containing an azido group substituent. The surface azide on labeled cells could then be reacted with FITC-conjugated cyclooctyne. The authors report that such a labeling method has no bearing on cell morphology and viability nor on exosomal size distribution and integrity of surface markers, hence exosomal targeting ability is preserved. The method offers the added advantage of sidestepping the difficulties associated with removal of membrane-unbound aggregates of conventional lipophilic dyes and minimizes background signals.
4.4. Exosomes biomimetics
Notwithstanding the demonstrable potential, many researchers have reservations regarding exosomes, especially with regards to their payloads compared to synthetic systems like liposomes. Although liposomes have a larger capacity for loading, exosomes afford better immunogenicity profiles, innate stability and higher efficiency of delivery (given their complex and natural membrane composition). In realizing the above, researchers have been recently working to develop exosome biomimetics - chimeric structures combining the best of both biological and synthetic vesicles. EXOPLEXs [53] stand as a perfect example of these. These newly reported structures are the product of membrane fusion of larger liposomes with those of contained exosomes, by extrusion. The produced EXOPLEX system can then loaded with the drug of choice. Even though the system has not been adequately validated, it offers a promising option to explore.
5. Theragnostic Exosomes
5.1. Exosomes in cancer
Exosomes of neoplastic origin, or “oncosomes”, seem to lie at the center of the insidious mechanisms tumors employ for gaining ground. By dint of an assortment of pro-oncogenic cargos, exosomes have been evinced to be key in EMT, malignant transformation, angiogenesis and immune evasion [54]. Studies of exosomes in cancer have been particularly insightful as to why specific patterns of metastasis are observed in certain types of tumors. Cell-type specific tropism has been shown to govern the “homing” of exosomes to their target tissues, by way of specific proteins in the exosomal membrane that constitute a recognition moiety of the recipient cell-surface markers [54]. Isolation of exosomes from peripheral tissues viz. blood or urine can easily provide a convenient platform for diagnosis by analyzing their transcriptome, proteome and even lipidome, which reflects the anomalous condition of their originating cells.
Stefsnius and colleagues [55] have demonstrated exosomal role as cancer initiators. They presented evidence that exosomes isolated from pancreatic cancer cells (capan-2) can initiate malignant transformation by haphazardly altering the genetic make-up of recipient cells. Injection of exosome-transformed cells into mice gave rise to aggressive neoplasms. Whereas co-incubation of capan-2-derived exosomes and fibroblasts - with or without pretreatment with the initiator 3-methylcholanthrene - yielded low oncogenic transformation, incubating the cells with capan-2 exosomes followed by tetradecanoyl phorbol acetate as promoter, showed a significantly increased transformation efficiency. Such an observation indicates that these exosomes act as initiators of malignant transformation by causing random mutations, yet are insufficient to bring about substantial changes to the cellular proteome.
CEP55, a protein associated with ALIX and the ESCRT complex and a known downstream target of the oncogene FOXM1, was exclusively found to be membrane-localized in exosomes derived from malignant head and neck squamous cell carcinoma cells [56]. Moreover, these exosomes were demonstrated to reprogram the transcriptome of recipient human normal oral keratinocytes. The expression profile was altered to pro-oncogenic effects.
Researchers from the University of Kentucky [57] adopted a hitherto uncommon approach for evaluating the diagnostic power of exosomes in non-small cell lung cancer (NSCLC). The study relied on lipid profiling of exosomes isolated from plasma, instead of looking into (the more conventionally researched) aberrant expression patterns of the exosomal proteome and/or miRNome. Sophisticated multivariate statistical clustering of the total exosomal lipidome revealed a panel that distinguished between early, late NSCLC patients and healthy controls.
Exosomes can be exploited for therapeutic purposes as well. By way of illustration, systemically administrable paclitaxel-loaded macrophage-derived exosomes for targeting pulmonary cancer metastases were recently established [58]. The formulation relied on surface biofunctionalization of the exosomes with a recognition moiety for targeting the sigma receptors overexpressed in pulmonary carcinoma. Interaction of the vectorising tag at the cell surface initiates receptor-mediated endocytosis of the exosomes, which showed outstanding accumulation in lung metastases and antineoplastic effects superior to those instigated by free paclitaxel or unmodified paclitaxel exosomes.
One ingenious way to tackle cancer is to stealthily introduce suicide genes that, upon internalization in the cancer cell, initiates destructive mechanisms that ends up killing the neoplasm from within. An endogenous modification of MSCs with the suicide fusion gene cytosine deaminase::uracil phosphoribosyl transferase of yeast, produced exosomes containing the mRNA for the gene [59]. The exosomes were taken up by the cancer cells on incubating the latter in the conditioned medium of the genetically engineered MSCs. On introducing the prodrug 5-fluorocytosine, the protein encoded by the mRNA within the internalized exosomes mediated the conversion of the 5-fluorocytosine to the active 5-fluorouracil. This strategy should be further investigated, with its promise in exclusively limiting cytotoxicity to cancer cells, given proper targeting.
Exosomes have been deployed as platforms for pulsatile drug delivery, oftentimes a propitious scenario in cancer chemotherapy. Wang et al. [60] adopted a dual ligand targeting strategy for a synergistic effect within the context of a photoresponsive exosomal system. Thanks to its amphiphilic nature, (1,2)-dioleoyl-sn-glycero-3-phosphoethanolaminepoly(ethylene glycol) (DSPE-PEG) was readily incorporated into the exosomal phospholipid bilayer. Culturing THP-1 macrophages with DSPE-PEG-SH and DSPE-PEG-RGD conjugates yielded cells functionalized with sulfhydryl groups and the integrin-binding RGD peptide. THP-1-derived exosomes exhibited both modifications. AuNRs constituted the next surface alteration, where it bound the sulfhydrylated exosomes by Au-S bonds. The bound AuNRs was made to link to folic acid, which was to serve as the second targeting moiety in cooperation with RGD. Beside its utility as a fatty acid anchor to exosomes, AuNRs served a more active role in the developing model. The study made use of AuNRs innate ability to absorb NIR and bring about a laser-induced thermal disruption of the exosomal membrane. Loading the photoresponsive, dually conjugated exosomes with doxorubicin yielded the chemotherapeutic, photothermal targeting system. The group confirmed successful targeting of tumor cells and remarkably improved cytotoxicity of NIR irradiated doxorubicin-containing exosomes compared with solely photothermal ablation-dependent therapy.
In lieu of a photoresponsive system, exosomes can be rendered responsive to the characteristically acidic pH of tumor tissues. In an exemplar study [61], the model cytotoxic doxorubicin was incorporated into exosomes along with anchorage of hyaluronic acid (HA) as a CD44 recognition moiety (CD44 is abundantly expressed on tumor cells), and a pH-responsive 3-(diethylamino)propylamine moiety. The engineered exosomes specifically targeted tumor cells by way of the HA tag and uptaken by receptor-mediated endocytosis. Collaterally, the extracellular tumor pH drop stimulated the release of the doxorubicin cargo.
Exosomes functionalized with superparamagentic nanoparticles (SPMNPs) models a mode of drug delivery via external stimuli. One such study [62] employed reticulocyte-derived transferrin receptor-exhibiting exosomes as a vehicle for cancer therapy. The exosomes were incubated with transferrin-labeled SPMNPs and loaded with doxorubicin. Following IV administration, the exosomes can be directed in vivo using an external magnet.
Exosomes displaying nucleolin, which is overexpressed in leukemia, have been utilized for detection of the malignancy with very high sensitivity [18]. Using magnetic beads labeled with anti-CD63, the exosomes are captured, whereby an aptamer recognizes and binds the surface nucleolin. An AuNP-DNA-FAM conjugate then hybridizes to the bound aptamer by way of its aptamer-complementary sequence. Subsequent use of a restriction endonuclease releases a DNA fragment with FAM, resulting in the de-quenching of fluorescence. The system was applied to exosomes from serum samples and was able to detect as few as 1×1012 exosomes per microliter of sample.
Given their implication in cancer dissemination and progression, an emerging therapeutic modality involves the inhibition of exosome biogenesis in donor cancer cells or preventing their uptake by recipient cells, exhibiting the cell type-specific tropism [54].
5.2. Exosomes in cardiovascular disease
Exosomes from the pericardial fluid were shown to contain miRNAs highly expressed in cardiomyocytes and proximal vasculature [63]. These exosomes enhanced the viability of endothelial cells and better attuned them for neovascularization. Moreover, pericardial fluid-derived exosomes restored blood flow in ischemic mouse models. Such proangiogenic capacity was attributed to an endogenous miRNA within endothelial cells, let-7b-5p, which was determined to be enriched in pericardial fluid exosomes. let-7b-5p was shown to target Transforming Growth Factor Beta Receptor 1 (TGFBR1) in endothelial cells to angiogenic outcomes. Pericardial fluid exosomes, enriched in let-7b-5p, downregulated TGFBR1 expression; hence the ability to enrich vasculature growth. Experimental silencing of exosomal let-7b-5p diminished their angiogenic activity, confirming the postulate that this particular myocardium-derived/endothelium-derived cargo, and the associated mode of action, is essentially the key factor at play.
Instead of pericardial fluid, Li et al. [64] investigated the angiogenic effects of coronary serum exosomes isolated from patients with myocardial ischemia on endothelial cells like HUVECs. In vitro testing revealed significantly higher endothelial cell migration, proliferation and tube formation following treatment with test exosomes compared to those isolated from normal individuals. Moreover, these exosomes stimulated circulation recovery and encouraged formation of vascular collaterals in a mouse hind-limb ischemic model, relative to control exosomes. The team suggested that hypoxia-stimulated cardiomyocytes, rather than cardiac fibroblasts or endothelial cells, are the source of coronary serum exosomes. To elucidate the mechanism underlying such proangiogenic role, total RNA from test and control exosomes were used for microRNA profiling. miR-939-5p was found to be significantly downregulated in test exosomes. Loss/gain of function studies showed that miR-939-5p inhibited iNOS expression. In consequence, endothelial NO production is reduced and angiogenesis is compromised.
miRNome profiling of MSCs-derived exosomes indicated enrichment of 23 miRNAs regulating more than 5000 genes involved in cardiovascular processes [65]. Mechanistic outlining of activity revealed miRNA-mediated regulatory circuits within the Wnt pathway as well as TGF-β and PDGF signaling-mediated fibrosis. Similar to pericardial fluid-derived and coronary serum-derived exosomes, the profiled exosome population showed antiapoptotic effects on cardiomyocytes and promoted angiogenesis in HUVECs. By the same token with which the TGFBR1-silencing let-7b-5p exerted its effects, miRNA panels in the tested exosomes decreased collagen outputs in fibroblasts. Concentrating their cargo of the identified miRNAs augmented exosome functionality. As it happens, having consistently demonstrated these effects, MSCs-derived exosomes are rapidly garnering illustrious repute with myocardial regeneration.
Vandergriff and associates [66] introduced infarct-targeting exosomes that could be administered intravenously with minimal off-target distribution. To that end, a cardiac homing peptide was conjugated to exosomes released by cardiosphere-derived cells (CDC) by way of a lipophilic linker that integrates well into the exosomal membrane. In vitro testing on neonatal rat cardiomyocytes (NRCMs) and H9C2 cells revealed that the tagged exosomes increased viability of serum-deprived NRCMs and decreased apoptosis in H2O2-challenged H9C2 cells. In vivo assessment of the exosomes in an ischemia/reperfusion rat model showed reduced infarct sizes, increased ejection volumes and improved angiogenesis and cardiac proliferation. The authors acknowledge that further investigation into the mechanisms by which CDC-exosomes mediate cardiac regeneration is needed. However, this among other studies demonstrate the promise of exosomes in tissue regeneration.
Exosomes with pro-angiogenic cargos can also have their origins in extracardiac yet still highly vascularized tissues. Intercellular transfer of these exosomes constitute one major route of homeostatic maintenance in hypoxic conditions and tumor angiogenesis. Exosomes isolated from the highly vascularized glioblastoma multiforme were shown to promote endothelial proliferation and tube formation in HUVECs and revascularization in a hind limb ischemia mouse model [67]. The effect was attributed to the microRNA, miR-221, which had been characterized and determined to be essential for tip cell migration. Among a panel of pro-angiogenic miRNAs, miR-221 was the most significantly upregulated. Moreover, the resident syndecan-4 and glypican-1 proteoglycans were postulated to play a cooperative role in reinforcing the angiogenic effects induced by miRNA-mediated gene regulation, via serving as co-receptors for the recognition and recruitment of extracellular pro-angiogenic factors, thereby initiating collateral signaling cascades.
A recently published study [68] aimed to develop a GMP10-compliant method for large-scale production of cardiac-progenitor cells-derived exosomes of clinical grade, and a number of start-ups are adopting similar exosomal systems as cell-free therapeutic platforms for myocardial infarction and acute coronary syndromes.
5.3. Exosomes in immunomodulation
Exosomes have displayed a dichotomous nature apropos of their immunological predilection. On the one hand, they have been demonstrated to contribute to immune evasion, promotion of infectivity and disease progression; on the other hand, exosomes have been established as primary players in immune response to multiple pathologies. Thanks to their position as a hub immune component, exosomes have evinced a valuable role in immunotheranostics, with infinitely wide range of applications.
To give a mere instance, miRNAs extracted from lung-derived exosomes unraveled their potential role in combating various strains of the influenza virus [69]. A singularly upregulated exosomal miR-483-3p induced the expression of interferon type I and pro-inflammatory cytokines in infected mice, accounting for the antiviral and inflammatory response of the containing exosomes.
A newly published cross-sectional multicenter study [70] identified two exosomal proteins as biomarkers for early diagnosis of T cell-mediated rejection in kidney transplant recipients. Among a candidate panel of 17 upregulated exosomal proteins, tetraspanin-1 and hemopexin were the most significantly upregulated in patients with T cell-mediated rejection and were thus proposed to be potential diagnostic biomarkers for acute T cell-mediated kidney graft rejection.
For all that, the clinical benefits of exosomes in transplant rejections go beyond detection. Exosomes isolated from human bone marrow-derived mesenchymal stem cells (hBMSCs) have been utilized as immunomodulators in islet transplantations [71]. The hBMSCs were transfected with Fas receptor siRNA (for enhanced insulin production and islet protection) and miR-375 inhibitor (to reverse the moderated compromise of cellular proliferation and insulin secretion). RT-qPCR revealed that the produced exosomes contained the transfected constructs (endogenous modification). Intravenous co-injection of hBMSCs and their exosomes (co-cultured with PBMCs) repressed islet apoptosis, T cell activity and ameliorated islet protection against inflammatory cytokines, in a dose-dependent manner.
A similar approach was adopted outwith of transplant immunorejection. The Journal of Molecular Therapy lately featured an article [72] describing the use of exosomes for delivery of small RNAs with the aim of modulating inflammatory response in lungs. Serum-derived exosomes were labeled and transformed with Myd88 siRNA (for silencing this component of the innate immunity), miR-15a mimic (for aggrandizing its TLR4-based anti-inflammatory effects) and miR-155 inhibitor (to counteract its pro-inflammatory effects). LPS-injected mice were made to inhale the engineered exosomes for intratracheal delivery and samples from bronchoalveolar lavage fluid were subsequently assessed. The exosomes were taken up by alveolar macrophages only; LPS-activated neutrophils failed to demonstrate successful uptake. The treatment was associated with a decline of pro-inflammatory cytokines and chemokines, a drop in the total count of macrophages and neutrophils and minimized cellular infiltration of pulmonary tissue.
5.4. Exosomes in neurological disorders
Exosomes are key contributors to the maintenance of homeostasis in the nervous system; they mediate two-way signal transduction between neurons and other glial cells, thereby upkeeping the finely coordinated physiological functions within the central nervous system. Failure in exosomal trafficking mechanisms is a major precipitator of neurological disorders [39].
As is the case with exosomes from other origins, neural cell-derived exosomes can be of diagnostic and therapeutic potentials. Given their nanoscale dimensions and consequent capability of crossing the BBB, exosomes can theoretically provide real-time insights to brain pathology. A recent collaborative effort by the University of Lodz in Poland [73] pursued transcriptomic profiling of serum exosomes in patients with relapsing remitting multiple sclerosis (RRMS). Four miRNAs were found to be differentially expressed in RRMS patients compared to healthy controls. The identified panel comprising hsa-miR-122-5p, hsa-miR-196b-5p, hsa-miR-301a-3p and hsa-miR-532-5p was significantly downregulated in serum exosomes of relapsing RRMS patients and such pattern was replicated in PBMCs from RRMS patients. Computational target prediction defined 74 putative mRNA sequences of the identified panel, most of which were involved in transcription and DNA interaction, suggesting a role of these microRNAs in regulation of gene expression. The results of this study indicate a likely utility of the defined panel as an RRMS signature.
Exosomal size is also advantageous in delivery of therapy to the brain. Exosomes obtained from naïve macrophages have been used for cargo delivery to inflamed brains [74]. Given their cells of origin, these exosomes exhibit an enrichment of intercellular adhesion molecule 1 (ICAM-1), which facilitates the uptake of these exosomes by BBB cells within the experimental inflammatory milieu. Elevation of lymphocyte function-associated antigen 1 (LFA-1) further supports this role and overexpression of C-type lectin receptors better equips these exosomes to interface with the endothelial barrier. The above-described exosomes delivered their cargo of brain-derived neurotrophic factor when administered intravenously. Given that most CNS conditions involve neuroinflammation on some level, the described approach of exosomal delivery should be extrapolatable to the preponderance of neurological disorders.
However, despite common disease features, response of disparate CNS pathologies to any generically tested disease-modifying modality is unpredictable; platforms that prove effective in one condition very often fail in biologically similar ones. A more focused approach, building on generic studies, while interrogating the utility of a developed platform on each individual disorder is more likely to determine its suitability and efficacy, with minimal inconsequential inferences, and would expedite progress toward clinically realizable outcomes.
Such efforts are progressively gaining ground and the accomplished upshots are edging their way to the clinic. One such study [75] explored exosomes as delivery vehicles in the context of Parkinson's disease. In this study, researchers loaded dopamine into unmodified blood exosomes by incubation in a saturated solution of the drug and tested the uptake of the loaded exosomes by mouse brain endothelial cells in vitro and the targeting ability of labeled exosomes in vivo. bEnd3 cells from the in vitro experiment manifested a time-dependent increase of exosomes uptake, while NIR fluorescent imaging of mice brains showed that exosomes accumulated selectively in the brain, following an IV injection. According to the authors, these dopamine loaded blood exosomes demonstrated a 15-fold improvement in dopamine brain distribution to free dopamine and significantly lower toxicity. The propounded system was also reported to be superior to levodopa, of which minuscule amounts reach the brain when administered systemically, thanks to the plasma abundant and PD brain-deficient levodopa decarboxylase11. The above results were further corroborated in a 6-OHDA PD model, with animals receiving the treatment showing a significant reduction in amphetamine-induced rotations12. The innate brain targeting abilities of blood exosomes was attributed to its surface transferrin receptors, which are brought to another set of transferrin receptors on microvascular endothelial cells by plasma transferrin. An earlier study [76] had designed a strategically different exosome-based therapeutic platform for PD. Building on the hypothesis that exosomes from monocytes/macrophages can escape immunosurveillance by mononuclear phagocytes; exosomes from culture media of macrophages - instead of blood exosomes - were isolated and loaded with the antioxidant catalase. The exosomes were taken up by neural cells in culture and detected in the 6-OHDA PD brain.
Intsead of exogenously provided exosomes, Kojima and colleagues [77] looked to deliver therapeutic mRNA cargos to parkinsonian-like brains of 6-OHDA-injected rats. To that end, they designed their “EXOsomal transfer into cells” (EXOtic) platform, by which they sought to genetically engineer the exosome-producing cells (endogenous modification-based approach). The EXOtic device comprised an exosome production booster composed of a tricistronic plasmid of genes determined to enhance exosome biogenesis. Making use of the exosomal surface marker, CD63, the gene encoding archaeal protein, L7Ae, which binds the C/D box of RNA, was conjugated to the sequence encoding the C-terminal end of CD63 to build a fusion protein that would simultaneously capture the C/D box of the mRNA of interest and package it in exosomes. A separate construct is included for the gene of interest (catalase). Two additional EXOtic devices are the constructs encoding the targeting peptide and a cytosolic delivery helper. Attenuation of neuroinflammation relative to the EXOtic-free group was detected in the tested animal models.
The EXOtic platform merits further investigation into the applicability in other diseases. Beyond maximizing therapeutic exosome release and controlling the delivery of intended biomolecules, sidestepping the need for exosome concentration is one advantage of EXOtic devices. Additionally, the steady supply of exosomes and consequent delivery of therapeutic biomolecules bypass the challenges associated with the short half-life of free (exogenously supplied) exosomes.
A study [78] published in Acta Neuropathologica as of late strongly suggests a central role for exosomes in the dissemination of Alzheimer's disease (AD) pathology. Instead of a prion-like spreading mechanism, the group defined exosomes as potential moderators of Aβ oligomers-mediated AD pathology propagation. The hypothesis was built on grounds of the endocytic degradation pathway involving Aβ aggregates. Post-mortem analysis of AD brains established association of the Aβ oligomers with exosomes. The incubation of exosomes isolated from such autopsies with cells in culture resulted in cellular uptake of the exosomes and their propagation to co-culture cells, with measurable neurotoxicity. Knockdown of key players (TG101 and VPS4A) in exosome biogenesis in donor cells and/or uptake in recipient cells halted such propagation, further proving the mechanistic involvement of exosomes. The authors suggest that phenothiazine-derived antipsychotics can be a proper therapeutic strategy for prevention of disease progression, given its surmised inhibitory effects on dynamin-mediated endocytosis, blocking uptake of the pathologic exosomes by healthy cells.
Considering the above, the use of exosome-associated Aβ oligomers for early detection of Alzheimer's disease has been trending and along with other targets is the subject of patents. A contemporarily declared technology used aptasensors for detection of picomolar concentrations of Aβ42 in blood of suspected AD subjects [79]. It is rationalized that the utility of the exosomes within the developed strategy stems from their ability to cross the blood brain barrier. Presence of exosomal Aβ42 in blood and concomitant absence of free Aβ42 in serum translates to early stages of AD, whereas a detectable Aβ42 signal in serum denotes later stages of the disease. Considering the sensitivity of the proposed method, absence of a signal entails complete absence of Aβ42, signifying a healthy state. The technology could potentially shift an established AD diagnosis 10-year earlier down its progression timeline. With the added advantage of antibody/peptides-independence, the assay has meritorious clinical prospects.
Beyond brain-targeted therapy, exosomes can be used for spinal cord injury as well. Exosomes retrieved from human umbilical cord mesenchymal stromal cells (hucMSCs) seem to be a promising treatment modality in spinal cord injury. As shown by Sun et al [80], hucMSCs-derived exosomes can potentially effect an M1 to M2 polarization shift in bone marrow-derived macrophages. In vivo experiments showed improved healing and amelioration of locomotor recovery on IV administration of huMSCs-derived exosomes, by curtailing levels of the proinflammatory cytokines, while boosting those of the anti-inflammatory mediators, suggesting the implication of exosomes in the innate immune response to injury.
With promising results from similar studies as outlined above, further investigative efforts comparing the developed platforms in the same experimental setting should be pursued to determine which of the developed systems, if any, can or should move on to pre-clinical testing.
5.5. Exosomes in locomotor disorders
Exosomes have been extensively researched as to their utility in muscloskeletal infirmities. A research article published in Stem Cell Research and Therapy [81] revealed a homeostatic role for exosomes in chondrocyte extracellular matrix. Exosomes derived from human embryonic stem cell-induced mesenchymal cells (ESC-MSCs) were found to mediate a balance between formation and degeneration of cartilaginous matrix. The study used IL-1β-treated primary mouse chondrocytes as an in vitro model. The cells were incubated with conditioned medium (CM) containing ESC-MSCs-derived exosomes and another group with exosome-free CM. Subsequent immunohistochemistry and western blotting analyses betrayed a notable dose-dependent increase in collagen type II with concomitantly decreased expression of the matrix degradation enzyme, ADAMTS5. ESC-MSCs-derived exosomes also prevented cartilage degradation in a destabilization of the medial meniscus (DMM) model following post-surgical intra-articular injection as demonstrated by substantially lower OARSI scores13 and immunohistochemistry results analogous to those obtained with IL-1β-treated chondrocytes.
Another endeavor [82] evaluated the involvement of bone marrow derived MSC-derived (BMMSC) exosomes on cartilage regeneration. BMMSC exosomes incubated with TNF-α stimulated osteoarthritis (OA) chondrocyte cells, instigated increased expression of COX-2, repressed TNF-α-promoted collagenase action and suppressed proinflammatory interleukins. BMMSC exosomes were also shown to upregulate the expression of genes vital in chondrogenesis (SRY-box9, SOX9 and Wnt7A) and downregulate others involved in hypertrophy (RUNX2, COL10A1 and ALP). Furthermore, BMMSC exosomes stimulated synthesis of proteoglycans and collagen type II in OA chondrocytes. The authors delineated that the mechanism of action by which BMMSC exosomes effect such anti-inflammatory outcomes involves inhibition of NFκB signaling, where TNF-α-mediated phosphorylation of IκBα (inhibitory subunit of NFαB) is blocked by BMMSC exosomes.
In addition to inflammatory arthritis and chondromalacia, some myopathologies are relatable to an exosomal component. For example, a study published in Human Molecular Genetics by Koutsoulidou and colleagues [83] defined a panel of four miRNAs; miR-1, miR-133a, miR-133b and miR-206 that was muscle tissue-specific and correlated with the progression of muscle atrophy in patients with Myotonic Dystrophy Type 1 (MD1), the typical form of late-onset muscle dystrophy in which muscle tissue progressively wastes away. The levels of these miRNAs were substantially higher in MD1 patients relative to the healthy and stable disease groups. The resistance of the defined miRNA panel to RNase in serum was attributed to its encapsulation in exosomes rather than association with shielding serum proteins as HDL, ApoA1 or Argonaut proteins. The authors accordingly extrapolated that these exosomal-derived miRNAs could serve as disease biomarkers in patients with Myotonic Dystrophy Type 1.
5.6. Exosomes in regeneration
The regenerative power of exosomes has been herein demonstrated in myocardial infarction as well as with degenerating cartilaginous tissues. However, exosomes have been shown to mediate tissue regeneration in various other contexts. Researchers at the oral biology and endodontics departments of the University of Illinois Chicago [84] used exosomes from dental pulp cells to induce in vitro and in vivo odontogenesis in naïve human dental pulp stem cells and hBMSCs. The exosomes were shown to bind components of the extracellular matrix (fibronectin and collagen type I) and subsequently endocytosed by the cells. The group reported the upregulation of genes involved in lineage specification of the odontogenic niche and an upswing in MAPK signaling in hBMSCs as well as 2D and 3D culture models of naïve human dental pulp stem cells. Additionally, the exosomes demonstrated regenerative effects in a tooth root slice model. Exosomes isolated under odontogenic conditions showed an enhanced differentiation capacity, marked by formation of the restorative dentin, which indicates that the condition of the parent cells is of essence to optimal functionality of the derived exosomes.
Instead of differentiating stem cells, Lee et al. [85] worked to reprogram differentiated fibroblasts to neural progenitor cells using exosomes they coined, “reprosomes”. These autologous reprogramming exosomes again exerted their effect on account of the contained medley of neural lineage specific factors. The authors report an outstandingly expeditious lineage conversion and maintenance of self-renewal for several weeks. The reprogramed neural progenitors subsequently differentiated to the distinct subclasses of neural cells. Similar to the work set-up from the dental pulp regeneration study, the fibroblasts were cultured in neural stem cell medium and further subjected to ultrasound, ahead of exosome isolation, to encourage the release of the necessary factors and subsequent packaging into the would-be isolated “reprosomes”.
The regenerative power of exosomes has been exploited in wound healing as well. Exosomes isolated from platelet-rich plasma contain a plethora of platelet-produced growth factors and can thereby mediate similar healing effects as platelet-rich plasma [86]. These exosomes promoted the reepithelization of non-healing wounds by enhancing migration and proliferation of endothelial cells as well as fibroblasts.
Exosomes isolated from the CM of stem cells retrieved from urine samples demonstrated significant healing effects on diabetic mice models [87]. The healing effect was attributed to the pro-angiogenic activity of the protein deleted in brain tumors 1 (DMBT1).
6. Exosomes in Action
With the groundbreaking potential of exosomes and other EVs, evinced by the exponential rise in the number of publications and the plenitude of promising results reported, it was only natural that these minuscule bearers of biological information found their way toward the clinic. The pursuit of bringing exosomes to the market was readily incentivized by their adaptability to engineering and the availability of data expounding their biology and isolation protocols, not to mention their intrinsic clinical value in terms of toxicity and immunogenicity profiles.
Such accruing interest is reflected in the number of issued patents and exosome technology-adopting start-ups. According to a recent report published in Frost & Sullivan [88], a total of 225 EV-related patents worldwide have been issued as of 2017, with the US at the forefront of the “global patent landscape”, followed by China. (Figure 5) outlines the segmentation of regional patenting activities.
The report also acknowledges a growth opportunity for EVs (of which exosomes are the cardinal subtype of clinical interest), specifically in the area of liquid biopsies. EVs are counted among the top three biomarkers (alongside ctDNA and CTCs) of the ever-growing diagnostic trend of liquid biopsies, which - according to the report - is anticipated to replace diagnostic tissue biopsies by up to 40% within 2019 [89].
6.1 Exopreneurs
The paradigm shift occasioned by continual breakthroughs with exosome research is already underway, and the market landscape is reshaping accordingly. Where interest in exosomes started as a purely scientific interest, introducing novel exosome-based platforms has become a race for supremacy. A number of start-ups have recently been cropping up, each with a unique portfolio and established proprietary technologies. Below, the most prominent of these are discussed, with the objective of illustrating how exosomes are already changing mainstream biomedicine.
Capricor Therapeutics
As a corollary to its proprietary cardiosphere-derived cells (CDCs), Capricor Therapeutics [90] recently established its first exosomal product, CAP-2003, which is currently a long way into pre-clinical testing. CAP-2003 represents exosomes isolated from the company's CDCs. CAP-2003 is expected to possess the anti-inflammatory, pro-angiogenic, antiapoptotic and antifibrotic effects associated with their parent CDCs, and thus is surmised to serve as a platform in regenerative medicine.
Kimera Labs
Kimera Labs [91] developed a state-of-the-art product for skin rejuvenation, which they named XoGlo™. XoGlo is a topical, placental MSC-derived exosome-based clinically available product that orchestrate tissue redipositioning, preventing healing-associated scarring.
Exosomedx
Exosome Diagnostics (Exosomedx) [92] developed a line of exosome-based diagnostic tests spanning a spectrum of conditions. Their proprietary ExoDx series is currently available in the clinic. In accordance with the Clinical Laboratory Improvement Amendments (CLIA) act of 1988, Exosomedx is certified to conduct advanced clinical testing.
Trends of regional patent issuance for EV technologies. The regions segmented based on the number of issued patents. Source: lens.org, Frost & Sullivan.
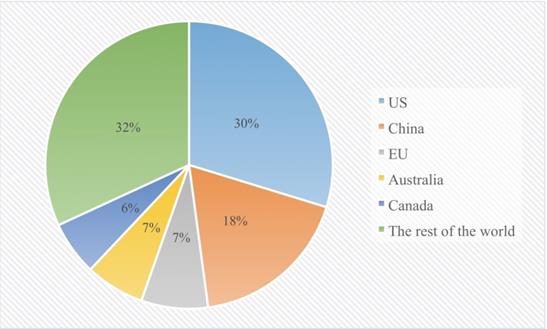
Their proprietary technology for exosomal RNA isolation from plasma/serum has been extended to research laboratories around the world through the exoRNeasy Serum/Plasma kits marketed by QIAGEN. Promega is to exclusively carry the Exosome Diagnostics' Urine Clinical Sample Concentrator Kit for concentration of urinary exosomes in clinical samples to make way for preps of high enough quality for molecular diagnostics. The kit shall be employed to process samples for clinical trials. Through their A to D (Academic Bench to Diagnostic Assay) program, Exosomedx offers partnership opportunity to researchers seeking to extrapolate their developed technologies to the clinic. In June 2018, it was announced that Bio-Techne was to acquire Exosomedx.
NanoSomiX
NanoSomix [93], a La Paz-based company, works within a CLIA laboratory environment to produce brain-derived exosomes (BDEs) from various neural cell populations. Their interest lies primarily in isolation of BDEs from peripheral tissues as disease biomarkers for prediction of potential nervous system disorders. Two assays relying on surface (ExoM) and core markers (ExoC) have been set forth. The company recently announced the issuance of their first US patent, entitled "Biomarkers and Diagnostic Methods for Alzheimer's Disease and Other Neurodegenerative Disorders". The patent concerns NanoSomix's proprietary BDE isolation technology and will be granting exclusivity to blood-based BDE diagnostic testing to NanoSomix until October 24, 2034. Five additional patents, concerning various aspects of the technology, are currently being considered.
Evox Therapeutics
Evox Therapeutics [94] is the product of collaborative efforts between the University of Oxford and Karolinska Institute. Evox works to develop exosome-based therapeutics whether for its pipeline or to other entities in partnership. Evox Therapeutics recently collaborated with Boehringer Ingelheim to apply the former's technology in delivering therapeutic RNAs pertinent to the latter's portfolio. According to published information, the partnership will seek to perform in-vitro and in-vivo studies using Evox state-of-the-art exosome research.
Evox employs both methods of endogenous and exogenous drug loading of exosomes and their proprietary pipeline is oriented at rare metabolic and lysosomal storage diseases such Niemann-Pick disease C, the proposed therapy of which is well on its way to clinical trials.
Evox Therapeutics has secured three patents protecting technologies for RNA loading, protein loading (whether luminal or surface) and tissue targeting. An additional patent filed for protection of the methodologies employed for exosomal isolation and characterization is still pending.
Codiak Biosciences
Combining their engEx™ platform and proprietary exosome purification technology, Codiak Biosciences [95] is one of the main industrial carriers of engineered therapeutic exosomes. They too employ both endogenous and exogenous methods of exosome loading, and are quickly moving toward the market with avant-garde exosome-based products. Their pipeline encompasses products within the immuno-oncology domain, vaccine adjuvants, anti-infectives, modulators of autoimmune disorders and anti-inflammatory therapeutics. Two of its proprietary product range have passed on from pre-clinical testing to IND procedurals. Moreover, three of its cancer immunotherapeutics are set for phase 1/2 clinical trials in 2020.
ArunA Biomedical
Through a number of preclinical studies, Aruna Biomedical [96] demonstrated the translational potential of exosome-based therapeutics in various murine thromboembolic models of stroke through their lead product AB126. Dubbing itself as “The neural exosome company”, ArunA Biomedical recently announced the launch of its exosome-based line of therapeutics that are inherently capable of crossing the blood brain barrier and are thus surmised to be of remedial value to a number of central nervous system and neurodegenerative disorders. According to a company spokesperson, ArunA Biomedical have set plans to begin their pilot study on humans in 2019.
Exocyte Therapeutics
Exocyte Therapeutics [97], a Singaporean biotech company, is pioneering exosome-based cancer vaccines. Their technology involves electroporating tumor-derived exosomes (containing oncogenic messages) isolated from patient's blood into autologous dendritic cells, which is administered back to the patient along with a checkpoint inhibitor. In vivo, the dendritic cells express the antigenic information encoded in the electroporated exosome. The expressed oncogenic epitopes are then recognized by the patient's T cells, thereby stimulating cytotoxic T lymphocytes to attack any cell exhibiting these epitopes. The exosome-transfected dendritic cells also release cytokines and chemokines that help recruit cytotoxic natural killer cells for a combined antineoplastic effect. Exocyte Therapeutics currently hold patents in the US, china and Japan, granting protection for the use of exosome-electroporated dendritic cells as a cancer vaccine. The first human clinical trial was set to begin in 2018.
7. Future Outlook
Exosomes are rapidly becoming recognized for their promise in shifting current paradigms of disease theranostics and are anticipated to maintain an ever rising trend toward a commercial drift, which is contemporarily evident with the advent of commercialized exosome-based platforms. Nonetheless, exosomes as an auspicious recourse in theragnostic practices merits a more communal recognition and would benefit from being cast in the public eye.
Awareness across the scientific community as to the nature of exosomes, the rewards they afford and areas in need of expanding on should be vehemently encouraged. Additionally, exosome research remains in need of resourceful sponsorships and further breakthroughs in the field will require fostering by major entrepreneurial consortia and key businesses in cooperation with governmental agencies and academic establishments. Such collaborative efforts would gear industry and academia toward realizing quantum leaps with exosome technology and are of utmost importance if its prospects in revolutionizing contemporary biomedicine is to be realized.
Abbreviations
6-OHDA: 6-hydroxydopamine (oxidopamine); AD: Alzheimer's disease; AuNP: gold nanoparticles; AuNR: gold nanorods; BALF: bronchoalveolar lavage fluid; BBB: blood brain barrier; BDEs: brain-derived exosomes; BMMSCs: bone marrow-derived mesenchymal stem/stromal cells; CDCs: cardiosphere-derived cells; CLIA: clinical laboratory improvement amendments; CM: conditioned medium; CNS: central nervous system; CTCs: circulating tumor cells; ctDNA: circulating tumor DNA; DMM: destabilization of the medial meniscus; EBV: Epstein-Barr virus; EGFP: enhanced green fluorescent protein; EMT: epithelial-mesenchymal transition; ESC: embryonic stem cells; ESCRT: endosomal sorting complexes required for transport; EVs: extracellular vesicles; EXOtic: exosomal transfer into cells; FAM: fluorescein; FITC: fluorescein isothiocyanate; GPI: glycosylphosphatidylinositol; HA: hyaluronic acid; hBMSCs: human bone marrow mesenchymal stem/stromal cells; HCV: hepatitis C virus; HIV: human immunodeficiency virus; hucMSCs: human umbilical cord mesenchymal stem/stromal cells; HUVECs: human umbilical vein endothelial cells; ILVs: intraluminal vesicles; IND: investigational new drug; iNOS: inducible nitric oxide synthase; LPS: lipopolysaccharide; MD: myotonic dystrophy; MDRCs: myeloid-derived regulatory cells; MSCs: mesenchymal stem/stromal cells; MUB: membrane-anchored ubiquitin; MVBs: multivesicular bodies; NIR: near-infrared; NMDEs: nasal mucosa-derived exosomes; NO: nitric oxide; NRCMs: Neonatal rat cardiomyocytes; NSCLC: non-small cell lung cancer; OA: osteoarthritis; OARSI: osteoarthritis research society international; PBMCs: peripheral blood mononuclear cells; PD: parkinson's disease; RISC: RNA-induced silencing complex; RRMS: relapsing-remitting multiple sclerosis; SPMNPs: superparamagnetic nanoparticles; TLR: toll-like receptor; UBL: ubiquitin-like.
Acknowledgements
A.A is the recipient of the AUC internal grant. M.Y is the recipient of the AUC Laboratory Fellowship.
Competing Interests
The authors have declared that no competing interest exists.
References
1. Beit-Yannai E, Tabak S, Stamer WD. Physical exosome:exosome interactions. J Cell Mol Med. 2018; vol. 22, no. 3. 2001 -2006
2. Colombo M, Raposo G, Théry C. Biogenesis, Secretion, and Intercellular Interactions of Exosomes and Other Extracellular Vesicles. Annu Rev Cell Dev Biol. 2014 vol. 30, no. 1: 255-289
3. Hanson PI, Cashikar A. Multivesicular Body Morphogenesis. Annu Rev Cell Dev Biol. 2012 vol. 28, no. 1: 337-362
4. Pathan M. et al. Vesiclepedia 2019: a compendium of RNA, proteins, lipids and metabolites in extracellular vesicles. Nucleic Acids Res. 2018 vol. 47, no. D1: D516-D519
5. Keerthikumar S. et al. ExoCarta: A Web-Based Compendium of Exosomal Cargo. J Mol Biol. 2016 vol. 428, no. 4: 688-692
6. Li S. et al. ExoRBase: A database of circRNA, lncRNA and mRNA in human blood exosomes. Nucleic Acids Res. 2018 vol. 46, no. D1: D106-D112
7. Kim DK. et al. EVpedia: a community web portal for extracellular vesicles research. Bioinformatics. 2015 vol. 31, no. 6: 933-939
8. Kourembanas S. Exosomes: Vehicles of Intercellular Signaling, Biomarkers, and Vectors of Cell Therapy. Annu Rev Physiol. 2015 vol. 77, no. 1: 13-27
9. Hessvik NP, Llorente A. Current knowledge on exosome biogenesis and release. Cell Mol Life Sci. 2018 vol. 75, no. 2: 193-208
10. Colombo M. et al. Analysis of ESCRT functions in exosome biogenesis, composition and secretion highlights the heterogeneity of extracellular vesicles. J Cell Sci. 2013 vol. 126, no. Pt 24: 5553-65
11. Jackson CE, Scruggs BS, Schaffer JE, Hanson PI. Effects of Inhibiting VPS4 Support a General Role for ESCRTs in Extracellular Vesicle Biogenesis. Biophys J. 2017 vol. 113, no. 6: 1342-1352
12. Schmidt O, Teis D. The ESCRT machinery. Curr Biol. 2012 vol. 22, no. 4: R116-20
13. Niel G van, D'Angelo G, Raposo G. Shedding light on the cell biology of extracellular vesicles. Nat Rev Mol Cell Biol. 2018 vol. 19, no. 4: 213-228
14. Bissig C, Gruenberg J. ALIX and the multivesicular endosome: ALIX in Wonderland. Trends Cell Biol. 2014 vol. 24, no. 1: 19-25
15. Nabhan JF, Hu R, Oh RS, Cohen SN, Lu Q. Formation and release of arrestin domain-containing protein 1-mediated microvesicles (ARMMs) at plasma membrane by recruitment of TSG101 protein. Proc Natl Acad Sci. 2012 vol. 109, no. 11: 4146-4151
16. Nickerson DP, West M, Odorizzi G. Did2 coordinates Vps4-mediated dissociation of ESCRT-III from endosomes. J Cell Biol. 2006 vol. 175, no. 5: 715-20
17. Hurley JH, Hanson PI. Membrane budding and scission by the ESCRT machinery: it's all in the neck. Nat Rev Mol Cell Biol. 2010 vol. 11, no. 8,: 556-566
18. Yang Z. et al. Structural Basis of Molecular Recognition between ESCRT-III-like Protein Vps60 and AAA-ATPase Regulator Vta1 in the Multivesicular Body Pathway. J Biol Chem. 2012 vol. 287, no. 52: 43899-43908
19. Trajkovic K. et al. Ceramide Triggers Budding of Exosome Vesicles into Multivesicular Endosomes. Science. 2008 vol. 319, no. 5867: 1244-1247
20. Kosaka N, Iguchi H, Yoshioka Y, Takeshita F, Matsuki Y, Ochiya T. Secretory Mechanisms and Intercellular Transfer of MicroRNAs in Living Cells. J Biol Chem. 2010; vol. 285, no. 23. 1744 2-17452
21. Li S, Lin Z, Jiang X, Yu X. Exosomal cargo-loading and synthetic exosome-mimics as potential therapeutic tools. Acta Pharmacol Sin. 2018 vol. 39, no. 4: 542-551
22. Gassart A de, Geminard C, Fevrier B, Raposo G, Vidal M. Lipid raft-associated protein sorting in exosomes. Blood. 2003 vol. 102, no. 13: 4336-4344
23. Wubbolts R. et al. Proteomic and Biochemical Analyses of Human B Cell-derived Exosomes. J Biol Chem. 2003 vol. 278, no. 13: 10963-10972
24. Staubach S, Razawi H, Hanisch FG. Proteomics of MUC1-containing lipid rafts from plasma membranes and exosomes of human breast carcinoma cells MCF-7. Proteomics. 2009 vol. 9, no. 10: 2820-2835
25. Dubois L, Ronquist KG, Ek B, Ronquist G, Larsson A. Proteomic Profiling of Detergent Resistant Membranes (Lipid Rafts) of Prostasomes. Mol Cell Proteomics. 2015 vol. 14, no. 11: 3015-3022
26. Andreu Z, Yáñez-Mó M. Tetraspanins in extracellular vesicle formation and function. Front Immunol. 2014 vol. 5, no. SEP: 1-12
27. Charrin S, Jouannet S, Boucheix C, Rubinstein E. Tetraspanins at a glance. J Cell Sci. 2014 vol. 127, no. 17: 3641-3648
28. Obata Y. et al. Adiponectin/T-cadherin system enhances exosome biogenesis and decreases cellular ceramides by exosomal release. JCI Insight. 2018 vol. 3, no. 8: 1-18
29. Sinha S. et al. Cortactin promotes exosome secretion by controlling branched actin dynamics. J Cell Biol. 2016 vol. 214, no. 2: 197-213
30. Gangoda L, Mathivanan S. Cortactin enhances exosome secretion without altering cargo. J Cell Biol. 2016 vol. 214, no. 2: 129-131
31. Ageta H. et al. UBL3 modification influences protein sorting to small extracellular vesicles. Nat Commun. 2018 vol. 9, no. 1: 1-3
32. Hough KP. et al. Exosomal transfer of mitochondria from airway myeloid-derived regulatory cells to T cells. Redox Biol. 2018 vol. 18: 54-64
33. Nocera AL. et al. Exosome swarms eliminate airway pathogens and provide passive epithelial immunoprotection through nitric oxide. J Allergy Clin Immunol. 2018 [Epub ahead of print]
34. Kulkarni R, Prasad A. Exosomes Derived from HIV-1 Infected DCs Mediate Viral trans-Infection via Fibronectin and Galectin-3. Sci Rep. 2017 vol. 7, no. 1, p. 14787
35. Nanbo A, Kawanishi E, Yoshida R, Yoshiyama H. Exosomes Derived from Epstein-Barr Virus-Infected Cells Are Internalized via Caveola-Dependent Endocytosis and Promote Phenotypic Modulation in Target Cells. J Virol. 2013 vol. 87, no. 18: 10334-10347
36. Cosset FL, Dreux M. HCV transmission by hepatic exosomes establishes a productive infection. J Hepatol. 2014 vol. 60, no. 3: 674-5
37. Giri PK, Kruh NA, Dobos KM, Schorey JS. Proteomic analysis identifies highly antigenic proteins in exosomes from M. tuberculosis-infected and culture filtrate protein-treated macrophages. Proteomics. 2010 vol. 10, no. 17: 3190-3202
38. Zhou W. et al. Exosomes serve as novel modes of tick-borne flavivirus transmission from arthropod to human cells and facilitates dissemination of viral RNA and proteins to the vertebrate neuronal cells. PLoS pathogens. 2018 vol. 14, no. 1: e1006764
39. Kawahara H, Hanayama R. The Role of Exosomes/Extracellular Vesicles in Neural Signal Transduction. Biol Pharm Bull. 2018 vol. 41, no. 8: 1119-1125
40. Sinha M. Sardar et al. Alzheimer's disease pathology propagation by exosomes containing toxic amyloid-beta oligomers. Acta Neuropathol. 2018 vol. 136, no. 1: 41-56
41. DeLeo AM, Ikezu T. Extracellular Vesicle Biology in Alzheimer's Disease and Related Tauopathy. J Neuroimmune Pharmacol. 2018 vol. 13, no. 3: 292-308
42. Rajagopal C, Harikumar KB. The Origin and Functions of Exosomes in Cancer. Front Oncol. 2018 vol. 8: 66
43. Ruivo CF, Adem B, Silva M, Melo SA. The Biology of Cancer Exosomes: Insights and New Perspectives. Cancer Res. 2017 vol. 77, no. 23: 6480-6488
44. Kim JH, Kim E, Lee MY. Exosomes as diagnostic biomarkers in cancer. Mol Cell Toxicol. 2018 vol. 14, no. 2: 113-122
45. Sun W, Luo J, Jiang H, Duan DD. Tumor exosomes: a double-edged sword in cancer therapy. Acta Pharmacol Sin. 2018 vol. 39, no. 4: 534-541
46. Kim YS, Ahn JS, Kim S, Kim HJ, S. Kim H, and Kang JS. The potential theragnostic (diagnostic+therapeutic) application of exosomes in diverse biomedical fields. Korean J Physiol Pharmacol. 2018 vol. 22, no. 2: 113-125
47. Biscans A. et al. Hydrophobicity of Lipid-Conjugated siRNAs Predicts Productive Loading to Small Extracellular Vesicles. Mol Ther. 2018 vol. 26, no. 6: 1520-1528
48. Haraszti RA. et al. Optimized Cholesterol-siRNA Chemistry Improves Productive Loading onto Extracellular Vesicles. Mol Ther. 2018; vol. 26, no. 8. 1973 -1982
49. Cheng Y, Schorey JS. Targeting soluble proteins to exosomes using a ubiquitin tag. Biotechnol Bioeng. 2016 vol. 113, no. 6: 1315-1324
50. Sterzenbach U, Putz U, Low LH, Silke J, Tan SS, Howitt J. Engineered Exosomes as Vehicles for Biologically Active Proteins. Mol Ther. 2017 vol. 25, no. 6: 1269-1278
51. Hung ME, Leonard JN. Stabilization of exosome-targeting peptides via engineered glycosylation. J Biol Chem. 2015 vol. 290, no. 13: 8166-8172
52. Wan S. et al. Molecular Recognition-Based DNA Nanoassemblies on the Surfaces of Nanosized Exosomes. J Am Chem Soc. 2017 vol. 139, no. 15: 5289-5292
53. Goh WJ. et al. EXOPLEXs: Chimeric Drug Delivery Platform from the Fusion of Cell-Derived Nanovesicles and Liposomes. Biomacromolecules. 2018 vol. 19, no. 1: 22-30
54. Syn N, Wang L, Sethi G, Thiery JP, Goh BC. Exosome-Mediated Metastasis: From Epithelial-Mesenchymal Transition to Escape from Immunosurveillance. Trends Pharmacol Sci. 2016 vol. 37, no. 7: 606-617
55. Stefanius K. et al. Cancer cell exosomes can initiate malignant cell transformation. bioRxiv. in press
56. Qadir F. et al. Transcriptome reprogramming by cancer exosomes: identification of novel molecular targets in matrix and immune modulation. Mol Cancer. 2018 vol. 17, no. 1: 97
57. Fan TWM. et al. Exosomal lipids for classifying early and late stage non-small cell lung cancer. Anal Chim Acta. 2018 vol. 1037: 256-264
58. Kim MS. et al. Engineering macrophage-derived exosomes for targeted paclitaxel delivery to pulmonary metastases: in vitro and in vivo evaluations. Nanomedicine Nanotechnology, Biol Med. 2018 vol. 14, no. 1: 195-204
59. Ursula A. et al. Prodrug suicide gene therapy for cancer targeted intracellular by mesenchymal stem cell exosomes. Int J Cancer. 2019 vol. 144, no. 4: 897-908
60. Wang J. et al. Designer Exosomes for Active Targeted Chemo-Photothermal Synergistic Tumor Therapy. Adv Funct Mater. 2018; vol. 28, no. 18. 1707 360
61. Lee H, Park H, Noh GJ, Lee ES. pH-responsive hyaluronate-anchored extracellular vesicles to promote tumor-targeted drug delivery. Carbohydr Polym. 2018 vol. 202: 323-333
62. Qi H. et al. Blood Exosomes Endowed with Magnetic and Targeting Properties for Cancer Therapy. ACS Nano. 2016 vol. 10, no. 3: 3323-3333
63. Beltrami C. et al. Human Pericardial Fluid Contains Exosomes Enriched with Cardiovascular-Expressed MicroRNAs and Promotes Therapeutic Angiogenesis. Mol Ther. 2017 vol. 25, no. 3: 679-693
64. Li H. et al. Coronary Serum Exosomes Derived from Patients with Myocardial Ischemia Regulate Angiogenesis through the miR-939-mediated Nitric Oxide Signaling Pathway. Theranostics. 2018; vol. 8, no. 8. 2079 -2093
65. Ferguson SW. et al. The microRNA regulatory landscape of MSC-derived exosomes: A systems view. Sci Rep. 2018 vol. 8, no. 1: 1-12
66. Vandergriff A. et al. Targeting regenerative exosomes to myocardial infarction using cardiac homing peptide. Theranostics. 2018; vol. 8, no. 7. 1869 -1878
67. Monteforte A. et al. Glioblastoma Exosomes for Therapeutic Angiogenesis in Peripheral Ischemia. Tissue Eng Part A. 2017 vol. 23, no. 21-22: 1251-1261
68. Andriolo G. et al. Exosomes From Human Cardiac Progenitor Cells for Therapeutic Applications: Development of a GMP-Grade Manufacturing Method. Front Physiol. 2018 vol. 9: 1169
69. Maemura T. et al. Lung-Derived Exosomal miR-483-3p Regulates the Innate Immune Response to Influenza Virus Infection. The Journal of Infectious Diseases. 2018 vol 217, no. 9: 1372-1382
70. Lim JH. et al. Novel urinary exosomal biomarkers of acute T cell-mediated rejection in kidney transplant recipients: A cross-sectional study. PLoS One. 2018 vol. 13, no. 9: e0204204
71. Wen D, Peng Y, Liu D, Weizmann Y, Mahato RI. Mesenchymal stem cell and derived exosome as small RNA carrier and Immunomodulator to improve islet transplantation. J Control Release. 2016 vol. 238: 166-175
72. Zhang D, Lee H, Wang X, Rai A, Groot M, Jin Y. Exosome-Mediated Small RNA Delivery: A Novel Therapeutic Approach for Inflammatory Lung Responses. Mol Ther. 2018 vol. 26, no. 9: 2119-2130
73. Selmaj I. et al. Global exosome transcriptome profiling reveals biomarkers for multiple sclerosis. Ann Neurol. 2017 vol. 81, no. 5: 703-717
74. Yuan D. et al. Macrophage exosomes as natural nanocarriers for protein delivery to inflamed brain. Biomaterials. 2017 vol. 142: 1-12
75. Qu M. et al. Dopamine-loaded blood exosomes targeted to brain for better treatment of Parkinson's disease. J Control Release. 2018 vol. 287: 156-166
76. Haney MJ. et al. Exosomes as drug delivery vehicles for Parkinson's disease therapy. J Control Release. 2015 vol. 207: 18-30
77. Kojima R. et al. Designer exosomes produced by implanted cells intracerebrally deliver therapeutic cargo for Parkinson's disease treatment. Nat Commun. 2018 vol. 9, no. 1: 1305
78. Sinha M. Sardar et al. Alzheimer's disease pathology propagation by exosomes containing toxic amyloid-beta oligomers. Acta Neuropathol. 2018 vol. 136, no. 1: 41-56
79. Zhou J, Meng L, Ye W, Wang Q, Geng S, Sun C. A sensitive detection assay based on signal amplification technology for Alzheimer's disease's early biomarker in exosome. Anal Chim Acta. 2018 vol. 1022: 124-130
80. Sun G. et al. hucMSC derived exosomes promote functional recovery in spinal cord injury mice via attenuating inflammation. Mater Sci Eng C. 2018 vol. 89: 194-204
81. Wang Y. et al. Exosomes from embryonic mesenchymal stem cells alleviate osteoarthritis through balancing synthesis and degradation of cartilage extracellular matrix. Stem Cell Res Ther. 2017 vol. 8, no. 1: 189
82. Vonk LA. et al. Mesenchymal Stromal/stem Cell-derived Extracellular Vesicles Promote Human Cartilage Regeneration In vitro. Theranostics. 2018 vol. 8, no. 4: 906-920
83. Koutsoulidou A. et al. Identification of exosomal muscle-specific miRNAs in serum of myotonic dystrophy patients relating to muscle disease progress. Hum Mol Genet. 2017 vol. 26, no. 17: 3285-3302
84. Huang CC, Narayanan R, Alapati S, Ravindran S. Exosomes as biomimetic tools for stem cell differentiation: Applications in dental pulp tissue regeneration. Biomaterials. 2016 vol. 111: 103-115
85. Lee YS. et al. Exosome-Mediated Ultra-Effective Direct Conversion of Human Fibroblasts into Neural Progenitor-like Cells. ACS Nano. 2018 vol. 12, no. 3: 2531-2538
86. Guo SC, Tao SC, Yin WJ, Qi X, Yuan T, Zhang CQ. Exosomes derived from platelet-rich plasma promote the re-epithelization of chronic cutaneous wounds via activation of YAP in a diabetic rat model. Theranostics. 2017 vol. 7, no. 1: 81-96
87. Chen CY. et al. Exosomal DMBT1 from human urine-derived stem cells facilitates diabetic wound repair by promoting angiogenesis. Theranostics. 2018 vol. 8, no. 6: 1607-1623
88. T. Group. Innovations in Liquid Biopsy Techniques for Cancer Management Liquid Biopsy Technologies Likely to Lead the Transformation of Cancer Profiling and Personalized Treatment Strategies. Frost & Sullivan. 2017
89. P. Technologies and C. Study. 2015 Global Survey on Liquid Biopsy Adoption Trends Learn the Promising Technologies and Bottlenecks for CTCs and ctDNA — A Voice of Customer Study. Frost & Sullivan. 2015
90. Capricor Therapeutics. [Online]. Available: http://capricor.com/. [Accessed: 05-Dec-2018].
91. Kimera Labs. [Online]. Available: https://kimeralabs.com/. [Accessed: 05-Dec-2018].
92. Exosome Diagnostics. Personalized Precision Healthcare. [Online]. Available: http://www.exosomedx.com/. [Accessed: 05-Dec-2018].
93. NanoSomiX Inc. [Online]. Available: http://www.nanosomix.com/technology. [Accessed: 04-Dec-2018].
94. Evox Therapeutics. [Online]. Available: https://www.evoxtherapeutics.com/. [Accessed: 05-Dec-2018].
95. Codiak Biosciences. Realizing the potential of exosome biology. [Online]. Available: http://www.codiakbio.com/#cellular-communicators. [Accessed: 05-Dec-2018].
96. ArunA Biomedical. [Online]. Available: https://www.arunabiomedical.com/. [Accessed: 05-Dec-2018].
97. ExoCyte Therapeutics. [Online]. Available: http://exocytetherapeutics.com/. [Accessed: 05-Dec-2018].
98. Friand V, David G, Zimmermann P. Syntenin and syndecan in the biogenesis of exosomes. Biol Cell. 2015 vol. 107, no. 10: 331-341
Footnotes
Tetraspanins: A family of conserved transmembrane proteins, with four membrane-spanning alpha helices and two extracellular domains. These proteins play roles in many cellular processes including trafficking of proteins. CD63, CD9, CD81 tetraspanins are enriched in exosomal membranes.
GPI: Glycosylphosphatidylinositol is a membrane glycolipid that serves as membrane anchor to many proteins. It associates with several proteins post-translationally, which may function as another sorting mechanism.
Syndecans/syntenin system: Syndecans are membrane-associated proteoglycans, whereas syntenin is a syndecan-interacting protein. Syntenin contains a PDZ domain, which constitutes the site of interaction with syndecan. The syndecan/syntenin system has been proven to play a key role in exosomal biogenesis [98].
Cortactin: a protein involved in cytoskeletal modification.
Invadopodia: Actin-rich membrane protrusions, capable of breaking down the extracellular matrix by producing proteases.
Rab proteins: Small GTPases of the Ras superfamily with roles in membrane and vesicular trafficking along the cytoskeleton, as well as vesicular biogenesis and membrane fusion.
Ixodes Scapularis: A hard-bodied parasitizing tick known to act as a vector for several infectious diseases of animals including seven human pathogens, such as Lyme disease.
Cre Recombinase: A bacteriophage P1-derived topoisomerase that can act as a site-specific recombinase. Cre recombinase catalyzes recombination of DNA flanking specific recognition sequences known as loxP sites. Mice engineered to carry loxP sites are known as floxed mice.
Ndfip1: A protein known to be a target for ubiquitination by the “Nedd4” family of ubiquitin ligases.
GMP: Good Manufacturing Practice
Levodopa decarboxylase: An enzyme that converts the prodrug Levodopa into its pharmacologically active form, Dopamine.
Amphetamine-induced rotations: An index-based test commonly used in the assessment of legions in unilateral models of Parkinson's disease. It relies on a dyskinetic rotational behavior incited upon administration of amphetamine, an indirect agonist of dopamine.
OARSI scores: A scoring histopathology-based system for assessment of osteoarthritis.
Author contact
Corresponding author: Anwar Abdelnaser, PhD, Institute of Global Health and Human Ecology, School of Sciences and Engineering, The American University in Cairo, AUC Avenue, P.O. Box 74, New Cairo 11835, Egypt, E-mail: anwar.abdelnaseredu; Tel: +20 226152905
Received 2019-3-4
Accepted 2019-3-25
Published 2019-7-24